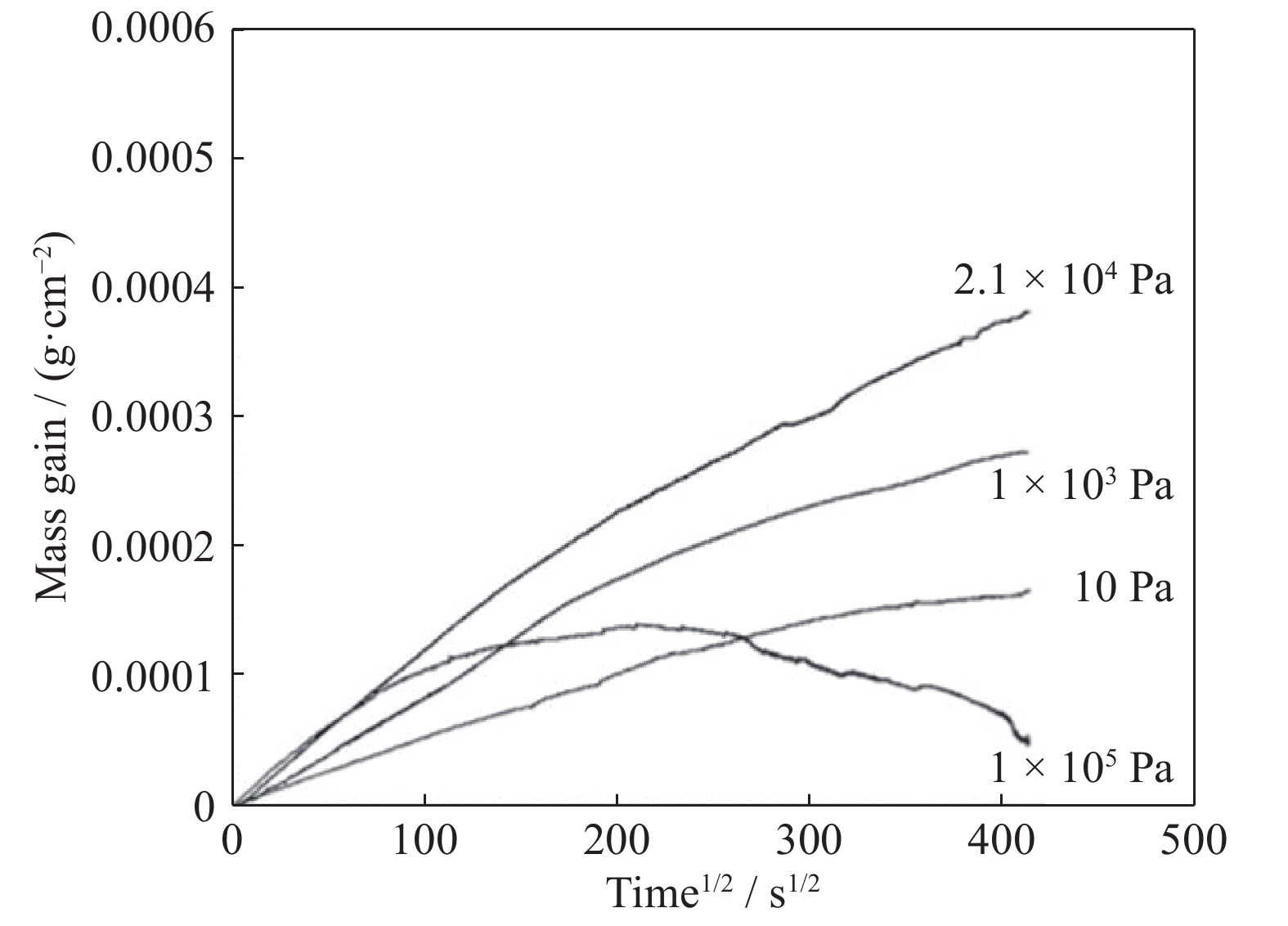
Cite this article as: | Ya Wei, Yu Fu, Zhi-min Pan, Yi-chong Ma, Hong-xu Cheng, Qian-cheng Zhao, Hong Luo, and Xiao-gang Li, Influencing factors and mechanism of high-temperature oxidation of high-entropy alloys: A review, Int. J. Miner. Metall. Mater., 28(2021), No. 6, pp.915-930. https://doi.org/10.1007/s12613-021-2257-7 |
High-temperature oxidation is a common failure in high-temperature environments, which widely occur in aircraft engines and aerospace thrusters; as a result, the development of anti-high-temperature oxidation materials has been pursued. Ni-based alloys are a common high-temperature material; however, they are too expensive. High-entropy alloys are alternatives for the anti-oxidation property at high temperatures because of their special structure and properties. The recent achievements of high-temperature oxidation are reviewed in this paper. The high-temperature oxidation environment, temperature, phase structure, alloy elements, and preparation methods of high-entropy alloys are summarized. The reason why high-entropy alloys have anti-oxidation ability at high temperatures is illuminated. Current research, material selection, and application prospects of high-temperature oxidation are introduced.
High-temperature oxidation refers to metal corrosion in which metal materials react with oxygen to form oxides at high temperatures. In the process of the high-temperature oxidation of metal, a complete and dense oxide film is formed that can have a protective effect on the metal. Superalloys have a wide range of applications, and the more common are Ni-based [1–3], which are mainly used in aerospace propulsion and aircraft engines. Cr2O3 and Al2O3 are common protective films for high-temperature oxidation processes. Studies have shown that Ni-based superalloys with Cr content greater than 30wt% have a protective effect [4]. Although nickel-based superalloys meet the requirements for use under high-temperature conditions [5–7], they are expensive and their use temperature limit is typically 1000°C. Price should not be ignored while pursuing the material performance. For Ni-based superalloys, the content of nickel is usually greater than 50wt%. Some high-entropy alloys, such as FeCoNiCrAl, perform well under high-temperature oxidation but are less expensive. Among pure metals with the same particle size and purity, the unit price of Fe, Co, Cr, and Al are all less expensive than Ni; therefore, high-entropy alloys have certain cost advantages and have emerged as alternatives.
High-entropy alloys have evolved from multi-principal alloy elements, which first appeared in Cantor’s undergraduate thesis [8], and high-entropy alloys were first introduced by Yeh et al. in 2004 [9]. High-entropy alloys generally comprise five or more alloy elements, and the content of each element is between 5at% and 35at%. The ideal mixed entropy of a high-entropy alloy is
Current research on the oxidation of high-entropy alloys is primarily divided into two categories, FeCoNiCr-based and refractory. FeCoNiCr-based studies mainly evaluate the effects of alloy elements, such as Al and Cr, on oxidation. Most of the early studies on high-entropy alloys are based on equal molar ratio; however, most researchers now use different element ratios to study the oxidation properties of high-entropy alloys, which can fully explore the specific role of alloy elements in the oxidation process and provide guidance for optimizing their composition. For refractory high-entropy alloys, the role of Mo, Ta, and other elements in the oxidation process is typically studied. The oxidation resistance of refractory metals is poor; however, with the deepening understanding of the high-temperature oxidation process, some high-entropy alloys with good oxidation properties have been identified.
This paper provides a specific discussion on recent research on the influence of high-entropy alloy high-temperature oxidation from the aspects of the service environment preparation process, phase structure, and alloy elements. By analyzing the influencing factors of high-entropy alloys, several mechanisms of oxidation of high-entropy alloys were obtained, and the future development of high-entropy alloys was proposed.
The FeCoNiCrMn high-entropy alloy was oxidized in different gas mixtures at 700 and 950°C. The higher the partial pressure of the CO2/CO gas mixture corresponds to the faster the oxidation rate at the same temperature. When the partial pressure of the CO2/CO gas mixture was the same, the higher the temperature was, the faster the oxidation rate was [44]. The oxidation kinetics curve of the Ni2FeCoCrAl0.5 high-entropy alloy is shown in Fig. 1 [45]. When the oxygen partial pressure was small, the oxidation rate increased with an increase in the oxygen partial pressure, and the oxidation weight kept increasing. A single α-Al2O3 layer was formed on the surface of the alloy, and its thickness increased as the oxygen partial pressure increased. When the oxygen partial pressure was 1.0 × 105 Pa, the oxidation weight gain increased at first and then decreased, which may be caused by the exfoliation of the oxide layer. At the oxygen partial pressure
The oxidation of HEA-1 (Al7.9Cr23.2Fe34.1Ni34.8), HEA-2 (Al8.9Cr23.1Fe33.7Ni34.3), HEA-3 (Al8.2Cr21.4Fe30.3Ni35Nb5.1), and HEA-4 (Al7.9Cr22Fe31.9Ni33.2Ti5) in a steam environment at 1200°C mainly came from the decomposition of water vapor [46]. The mass increases after oxidation are shown in Table 1, and the oxidation weight gain of HEA-4 was the greatest, indicating that the antioxidant performance of HEA-4 was the worst. Although HEA-4 contained Al, there was no Al2O3 protective film formed during the oxidation process, so the oxidation performance of HEA-1 to HEA-3 was better than that of HEA-4. After oxidation, HEA-4 formed a multi-layer oxide film, and internal oxidation occurred, resulting in a decrease in antioxidant performance [46].
HEA-1 | HEA-2 | HEA-3 | HEA-4 |
0.39 | 0.24 | 0.72 | 2.66 |
Generally, the higher the oxidation temperature, the faster the oxidation rate of the alloy. A higher temperature corresponds to a greater slope of the oxidation parabola, indicating that the oxidation rate is faster [47]. The kinetic curve of the oxidation behavior of the Ni2FeCoCrAlx high-entropy alloys in dry air at 600–900°C is similar to a previous report [48]. However, there are also special cases, such as CoCrFeMnNbxNi (x = 0 and 0.25, recorded as Nb0 and Nb0.25, respectively) [49]. At low temperatures, the antioxidant capacity of Nb0.25 is better than that of Nb0; however, its antioxidant capacity is worse than that of Nb0 at 900°C. The reason for this phenomenon illuminates the effect of the Nb element on high-temperature oxidation.
The high mixing entropy of high-entropy alloys contributes to the formation of solid solutions and inhibits the formation of intermetallic compounds. Yang and Zhang [50] summarized the law of phase formation by proposing a new parameter
Through the calculation of 20Mo–20W–20Al–20Cr–20Ti in the range of 200–2000°C, only the body-centered cubic (bcc) phase can exist stably at high temperature [51], as shown in Fig. 2(a). Pacheco et al. [52] found a similar rule in the study of HfNbTiVZr, as shown in Fig. 2(b), which provides guidance for the selection of materials for the study of oxidation of high-entropy alloys. A stable phase of bcc or materials converted to bcc at high temperatures should be chosen for oxidation experiments. With the addition of Al, the microstructure of the alloy changes from a face-centered cubic (fcc) phase to a mix of fcc phase and bcc phase. When the Al content continues to increase, the bcc structure of high-entropy alloy will continue to increase [53–57]. Uporov et al. [58] also determined that the bcc phase increases with the anneal time of the AlCoCrFeNiMn high-entropy alloy.
Al0.7CoCrFeNi alloy with Y/Hf has excellent high-temperature oxidation resistance. The addition of elements can inhibit defects and reduce segregation. Fewer interface defects can reduce the oxidation rate during oxidation, thereby improving oxidation resistance [59]. An extremely low oxidation rate and strong interfacial bonding make the Y/Hf-doped high-entropy alloy a promising high-temperature oxidation resistance material [60].
(1) Effect of the Al element on high-temperature oxidation.
The isothermal oxidation curve of the AlxCr0.4CuFe0.4MnNi high-entropy alloy at 800°C consists of two stages [61]. The first part follows the oxidation parabola law: the oxidation is mainly controlled by solid state diffusion, and the second part is the interface control process controlled by linear oxidation.
Experiments were conducted on the oxidation behavior of the AlxCr0.4CuFe0.4MnNi high-entropy alloy, and scanning electronic microscopy (SEM) images of the cross section of Cr0.4CuFe0.4MnNi alloy without Al were obtained, as shown in Fig. 3(a). The components of the corresponding points are shown in Table 2. The thickness of the oxide film is approximately 20 μm. Mn is oxidized preferentially because of less Cr, and the oxide film formed is not protective. The content of Cr in the alloy is limited (9.3at%). Hence, the protective layer of the alloy system is thin and the oxidation rate is high. The outermost oxide film is composed of a large number of Cu, Ni, and Mn, and some of the oxides are easy to peel off. Fig. 3(b) shows an SEM cross-sectional image of an Al0.3Cr0.4CuFe0.4MnNi alloy containing Al for comparison, and the components of the corresponding points are shown in Table 2 [61]. The oxide scale is only approximately 7 μm, indicating that the antioxidant capacity has been greatly improved with the addition of Al. Energy dispersive spectrometer (EDS) analysis shows that the innermost layer of the oxide film is a thin and dense protective layer of Cr and Al oxides; the rest is mainly rich manganese oxides. The contents of Ni and Cu in the outermost oxide film are very low, and the spalling phenomenon is still observed. The typical microstructure shows that manganese oxide has a negative effect on oxidation resistance because manganese oxide is unprotected and easy to peel off. The oxidation resistance of the high-entropy alloy is improved with the addition of Al because of the formation of Al2O3 [62–63].
Point | Fe | Cr | Ni | Mn | Cu | Al | O |
A (Cr + Mn OL) | 0.3 ± 0.1 | 3.4 ± 0.2 | 0.2 ± 0.1 | 42.8 ± 0.3 | 0.2 ± 0.1 | 0 | 53.4 ± 0.3 |
B (Mn OL) | 0.2 ± 0.1 | 0.2 ± 0.1 | 0.2 ± 0.1 | 51.1 ± 0.2 | 0.2 ± 0.1 | 0 | 48.1 ± 0.3 |
C (Mn + Cu + Ni OL) | 0.2 ± 0.1 | 0.2 ± 0.1 | 1.9 ± 0.2 | 45.8 ± 0.4 | 15.3 ± 0.2 | 0 | 37.0 ± 0.4 |
D (Cr + Al + Mn OL) | 0.2 ± 0.1 | 1.2 ± 0.1 | 0.2 ± 0.1 | 47.1 ± 0.1 | 0.2 ± 0.1 | 6.1 ± 0.1 | 44.3 ± 0.3 |
E (Mn OL) | 0.1 ± 0.1 | 0.3 ± 0.1 | 0.2 ± 0.1 | 45.3 ± 0.3 | 0.2 ± 0.1 | 0.3 ± 0.1 | 53.7 ± 0.4 |
F (Mn + Cu + Ni OL) | 1.6 ± 0.1 | 0.1 ± 0.1 | 0.8 ± 0.1 | 54.8 ± 0.3 | 1.4 ± 0.1 | 0.3 ± 0.1 | 41.7 ± 0.3 |
Note: OL—Oxide layer. |
The valence electron concentration (VEC) has a great influence on the crystal structure:
VEC=∑ni=1ci(VEC)i, |
where
The oxidation behavior of AlxCoCrCuFeNi at high temperatures was studied [66]. The interface between matrix and oxide film is no longer smooth after oxidation of Al0CoCrCuFeNi at 1000°C for 100 h because Al0CoCrCuFeNi has Cr without Al, so it is difficult to form a dense oxide film. According to our previous discussion, the outer layer is composed of hollow, loose Cr2O3 with poor adhesion. Unlike the Al0CoCrCuFeNi high-entropy alloy, the oxide layer and element distribution of the Al0.5, Al1.0, Al1.5, and Al2.0 high-entropy alloy vary with the increase in Al composition. The distribution of alloy elements becomes more uniform with increasing Al content, and the formed Al2O3 film becomes denser. Therefore, the oxidation resistance becomes better.
The continuity of Al2O3 becomes better with an increase in Al content; however, when the x of Alx(NiCoCrFe)100−x is less than 15, the oxidation rate increases significantly at the initial stage of oxidation. The Al2O3 formed with low Al content is discontinuous, thus reducing the antioxidant activity [67–68].
Ye et al. [69] found that the oxide film is mainly divided into three layers through the study of AlxCoCrFeMnNi. When Al is not added, the oxidation is very serious. When the Al element is added, a dense aluminum oxide protective film is formed, and the total oxide film thickness is reduced, indicating that the Al element improves the oxidation resistance. The EDS scan of the oxide film in Fig. 4 shows that the Mn content in the oxide film is gradually reduced, and the Al content is gradually increased. As shown in Fig. 4(e), at point 13, the Al content is 29.49at% and the Mn content is 27.08at%. At point 15, the Al content is 33.42at% and the Mn content is 4.76at%. The dense alumina formed during the oxidation prevents the diffusion of Mn elements, which reduces the Mn content of the inner layer and improves the oxidation resistance.
Recent studies have also shown that high-entropy alloys without Al have good high-temperature oxidation resistance. Through the comparative study of MoTaTiCrAl and MoTaTiCr, Li et al. [70] found that MoTaTiCr without Al forms a continuous CrTaO4 layer in the process of high-temperature oxidation, which plays a protective role in the process of high-temperature oxidation and makes the high-temperature oxidation resistance of MoTaTiCr better than that of MoTaTiCrAl. A continuous CrTaO4 layer can also effectively inhibit the volatilization of Mo, which is also the reason for the improvement of the antioxidant capacity of MoTaTiCr. This discovery also contradicts the traditional belief that adding Al can improve the antioxidant capacity at high temperatures, which is worthy of attention in future research.
(2) Effect of Ti element on high-temperature oxidation.
Ti can affect the oxidation properties of high-entropy alloys containing Al. Among the constituent elements of the CoCrFeNiAlxTiy [71] alloy system, Al has the highest affinity for oxygen. In the oxidation process, Al forms Al2O3, the most stable oxide in the CoCrFeNiAlxTi alloy. The weight gain of a high-entropy alloy is greater with the increase in Ti content. Although CoCrFeNiAlTi0.5 has a high content of Al, it does not show good antioxidant activity. The oxidation behavior of the alloy does not conform to the parabolic oxidation law. The oxidation process changes nearly linearly, indicating that the oxidation rate is very large, which shows that the oxide layer may peel off and the alloy may be oxidized again. Compared with other high-entropy alloys, the content of Ti in this alloy is the highest. As shown by the phase distribution below the oxide layer, Al is oxidized at the very beginning. A large amount of TiO2 is formed above this region and a Cr2O3 phase is formed on the TiO2 oxide film. In addition, a thin Al2O3 oxide film is formed on top of the internal alumina oxide. X-ray diffraction (XRD) analysis shows that there is a Fe2TiO5 phase in the initial stage after oxidation for 5 h, which may be formed by the reaction of Fe2O3 and TiO2. The phase is not clearly observed in the elemental atlas and scanning electron microscopy, which may be because the phase is unstable and difficult to capture. In the following stages, a large weight change at the end of the oxidation test also reveals the existence of this phase, which is unstable and grows rapidly. The increase in oxidation rate is because of the presence of Al2O3 oxide film. Ti ions will diffuse through Al2O3, which promotes the formation of oxides, resulting in the decrease in oxidation resistance of high-entropy alloys containing Ti.
Ham et al.’s research on AlCoCrFeNiTix [72] shows that Ti can improve the adhesion of Al2O3. As shown in Fig. 5, through the observation of the oxidation surface, both materials have Al2O3 oxides. The components of the corresponding points in Fig. 5 are listed in Table 3. The Ti0.0 material shows exfoliation of Al2O3 oxide (yellow area) in most areas, and the adhesion between Al2O3 and the high-entropy alloy is very low. The Ti1.0 alloy only exfoliates Al2O3 oxide in some areas and has better adhesion to Al2O3 than the Ti0.0 alloy. According to these results, adding a small amount of Ti to an AlCoCrFeNi high-entropy alloy can improve the adhesion of the oxide. Therefore, when we design high-entropy alloy materials containing Ti, we should consider the dual factors that Ti improves the adhesion ability of oxides and leads to a decline in oxidation resistance of high-entropy alloys to produce better oxidation resistance materials.
CoCrCuFeNi is a fcc solid solution and CoCrCuFeNi–TiO is composed of a fcc solid solution and TiO crystals. When a specific amount of TiO is added, TiO2 is formed during the oxidation process, which improves the oxidation resistance. However, with the increase in TiO, the precipitation of TiO2 leads to a decrease in oxidation resistance [73].
(3) Effect of Cu element on high-temperature oxidation.
The AlCoCrCuxFeNi [74] alloy was oxidized for 100 and 500 h, and the morphology of the obtained oxide film was evaluated by backscattered electron (BSE), as shown in Fig. 6. As shown in the oxidation kinetics curve, the spallation of two high-entropy alloys AlCoCrCu0.5FeNi and AlCoCrCuFeNi containing Cu is more serious, and the spallation is more obvious with the increase in Cu content. However, the
(4) Effect of Cr element on high-temperature oxidation.
The role of Cr in the oxidation process of high-entropy alloy FeCrxCoNiB was recently studied [75]. With the increase in Cr content, the grain size of the high-entropy alloy decreases, indicating that Cr can inhibit coarsening, which is also an important reason for the improvement in oxidation resistance of the high-entropy alloy. Boride is also easily oxidized at high temperatures to increase the oxidation trend. The increase in Cr will reduce coarsening and form dense Cr2O3, reducing the contact between oxygen and boron to improve the oxidation resistance in the oxidation process.
Al content can cause the "third element effect", increase the activity of Cr, and promote the formation of protective Cr2O3. In high-entropy alloys, once a continuous Cr2O3 layer is formed, the oxidation will be hindered. In the future alloy design, the activities of Cr and Al should be considered to form protective oxides more quickly [76].
(5) Effect of Mn element on high-temperature oxidation.
H5M (FeCoNiCrMn) high-temperature oxidation was studied at different temperatures [77]. Although there are some microcracks at the oxide film/substrate interface, especially at 900°C, the oxide film still has a good bond with the substrate. At 700, 800, and 900°C, the thickness of the oxide film of H5M is (10.17 ± 0.53), (13.61 ± 1.44), and (19.24 ± 0.87) μm, respectively. The serious oxidation situation shows that the thickness of the oxide film of H5M increases gradually after oxidation at different temperatures for 48 h. The Mn element forms MnO at the initial stage of oxidation and transforms into Mn3O4 in the later stage of oxidation. The outward diffusion rate of Mn ions is much faster than other elements, resulting in the formation of porous Mn films with binary and ternary oxide films on top of the Cr2O3 layer. Fig. 7 shows the energy dispersive X-ray spectroscopy (EDX) element diagram of the CrMnFeCoNi high-entropy alloy oxidized at 900°C for 100 h; qualitatively similar element distribution is observed [78]. The enrichment of Mn and Cr in the oxide film is serious. The oxide film is divided into two layers because of the different diffusion rates of the elements. The enrichment of Mn in the outer layer shows that the diffusion rate is greater than that of Cr. As the temperature increases, α-Mn2O3 gradually transforms into Mn3O4, forming pores near the oxide interface and reducing the oxidation resistance.
(6) Effect of Si element on high-temperature oxidation.
The oxidation kinetics of Nb–Mo–Cr–Ti–Al and Nb–Mo–Cr–Ti–Al–1Si shows that the oxidation rate containing Si is significantly less than those without Si at high temperatures greater than 900°C. When the temperature reaches 1000 and 1100°C, the oxide layer rich in Cr and Al is formed in Nb–Mo–Cr–Ti–Al–1Si alloy. Compared with the alloy without Si, the oxide layer rich in Cr and Al in the Nb–Mo–Cr–Ti–Al–1Si alloy is also compact. Therefore, the formation of Al-rich oxide film in the Nb–Mo–Cr–Ti–Al alloy system is affected by the amount of Si added [79].
When the Si content exceeds 0.6at%, the effect of Si on the anti-oxidation weakens [80]. The addition of Si leads to a decrease in the lattice constant, which leads to lattice distortion. Decreasing the lattice parameters will reduce the volume diffusion of oxygen or cations, thus reducing the degree of oxidation. However, with the Si content increasing in the cooling process, a hard-brittle composite phase (σ) appears, resulting in higher porosity. In addition, the lattice parameters of all of the constituent phases decrease with the increase in Si content, which may lead to stomata during the synthesis process. The role of Si slows anion diffusion and contributes to the infiltration of oxygen. This phenomenon leads to a decrease in anti-oxidation when the content of Si is too high, which causes the best antioxidant performance at 0.6at% Si.
The addition of Si will decrease the antioxidant performance. As shown in Fig. 8, in Si-free alloys, relatively small Laves phase particles are preferentially formed at grain boundaries. In the Si alloys, Laves phase particles are obviously larger and uniformly distributed in the matrix. The appearance of internal corrosion after high-temperature oxidation depends largely on the size, shape, and number of Laves phases (Fig. 9), which means that the matrix near the large-size Laves phase particles is more seriously eroded [81]. Wang et al. [82] came to a similar conclusion.
(7) Effect of Nb element on high-temperature oxidation.
In the high-temperature oxidation process, Nb plays a dual role in the Nb element in CoCrFeMnNbxNi: the new Laves phase hinders the outward diffusion of metal ions and also becomes a channel for the inward diffusion of oxygen ions. The combined effect of these two factors will lead to resistance oxidation fluctuations with changes in temperature, which supports why the antioxidant capacity of Nb0.25 is better than that of Nb0 at low temperature and worse at high temperature [49]. The high-temperature oxidation resistance of the Al0.2Co1.5CrFeNi1.5Ti0.3 high-entropy alloy is significantly improved after adding a trace amount of Nb [83]. During the oxidation process, a 4-μm thick Nb-rich layer is formed between Cr2O3 and Al2O3, which acts as a diffusion barrier, avoids internal diffusion of oxygen, and greatly improves high-temperature oxidation resistance.
(1) Effect of Zr and Hf elements on high-temperature oxidation.
Compared with Fig. 10(a), Fig. 10(b) shows that there is obvious internal oxidation [84]. The enrichment and dissolution of oxygen on the surface lead to an increase in the lattice constant, resulting in volume expansion and internal stress. As the oxidation process progresses, stress begins to accumulate; after reaching a certain level, the stress releases and causes fracture. Hf and Zr play an inseparable role in accelerating oxidation because Zr and Hf have high oxygen affinity and a large atomic radius, which leads to the weakening of interatomic bonds and an increase in intermetallic diffusion coefficient at high temperatures. Therefore, it is easier for oxides to form and cause the material to peel off.
(2) Effect of Mo element on high-temperature oxidation.
The oxide film of high-entropy alloy TiNbTa0.5ZrAlMo0.5 containing Mo becomes weaker and separates from the matrix during oxidation at 1000°C [85]. As shown in Fig. 11(a), vertical cracks are observed through the oxide film, which leads to the diffusion of oxygen into the substrate. A large number of nanoscale pores are observed around the cracks. The dispersed phase with the same pore size is molybdenum-rich oxide. According to the XRD results, the molybdenum-rich oxide is MoO3. Fig. 11(e) shows the subsurface of the oxide scale, with a total of three layers: the first layer contains many pores and defects, the second layer shows a transition oxide, as well as dispersed MoO3 and many nano-sized pores, and the third layer is a Mo-rich defect layer. The oxide scale of the TiNbTa0.5ZrAlMo0.5 alloy is closely related to MoO3. The melting point of MoO3 is the lowest (1000°C), which leads to the liquefaction of oxides at high temperatures. MoO3 has a high saturated vapor pressure and fast evaporation rate. Therefore, the large number of nanopores are considered evidence of MoO3 evaporation, which leads to the decrease in oxidation resistance. Waseem and Ryu [86] also confirmed that the oxidation resistance of high-entropy alloys containing Mo decreases because of evaporation.
(3) Effect of Ta element on high-temperature oxidation.
The microstructure of Ta–Mo–Cr–Ti–Al is analyzed in Fig. 12 [87]. After oxidation of the Ta–Mo–Cr–Ti–Al high-entropy alloy, the outer layer forms a continuous TiO2 layer and a continuous CrTaO4 layer at the bottom; however, the Cr2O3 and Al2O3 particles are discontinuous. TiO2 has limited protective ability, so CrTaO4 is the main reason for its high oxidation resistance. During the growth of CrTaO4-based oxides, a large number of elements are dissolved, which potentially inhibits these elements from reacting with oxygen and improves the oxidation resistance [88]. Lo et al. [89] also confirmed the antioxidant capacity of CrTaO4.
The high-temperature oxidation process of high-entropy alloys is most commonly performed in a vacuum arc furnace [90–91]. High-entropy alloys are also prepared by mechanical alloying [92–93], laser cladding [68,94], magnetron sputtering [95–99], and 3D printing [100]. High-entropy alloys for high-temperature oxidation research are generally vacuum arc furnace smelting or laser cladding. The oxidation of high-entropy alloys in vacuum arc smelting is outside the scope of this review.
The pre-oxidation conditions of the traditional thermal spraying of a MCrAlY (M = Ni, Co, Ni, and Co) bonding layer has high-speed laser cladding [22]. Compared with traditional thermal spraying, the high-entropy alloy bonding layer of high-speed laser cladding forms a denser aluminum oxide protective film during the oxidation process, which improves the high-temperature oxidation resistance. The non-bonding interface from thermal spraying causes the Al element to diffuse slowly, which does not form a continuous aluminum oxide protective film, reducing the oxidation resistance.
The oxide binding energy and electronegativity of elements are very important for the high-temperature oxidation of high-entropy alloys because it is related to the order of oxide formation. If the oxidation resistance of the oxides formed at first is good, it is possible to prevent oxygen from continuing to react with other alloy elements, thus improving the oxidation resistance of high-entropy alloys. If the oxidation resistance of the oxides formed before is relatively poor, it will accelerate the reaction of oxygen with other alloy elements, thus worsening the oxidation resistance of high-entropy alloys. For example, Fig. 13 shows the relationship between the oxide binding energy of the Al0.3CoCrFeNiCu high-entropy alloy and the electronegativity of elements [101]. For metallic materials, the fewer valence electrons, the greater the tendency of oxidation because it is easier to break away from the parent atom. Therefore, for the Al0.3CoCrFeNiCu high-entropy alloy, Al and Cr have fewer valence electrons and lower binding energy. Al2O3 and Cr2O3 are first formed to cover the surface of the matrix metal during high-temperature oxidation, which improves the oxidation resistance.
The formation of oxide film during high-temperature oxidation is also closely related to Gibbs free energy. Generally, oxides with lower Gibbs free energy are preferentially formed during oxidation. The Gibbs free energy during the oxidation process of common elements is shown in Table 4.
The Gibbs free energy shows that the order of oxide formation during oxidation is Al2O3 > SiO2 > MnO > Cr2O3 > FeO > CoO > NiO; Al is considered to have excellent high-temperature oxidation resistance during high-temperature oxidation. The Gibbs free energy of Mn is also relatively low and easy to form during the oxidation process. However, the oxide of Mn is usually relatively loose, so high-temperature oxidation resistance is not very good.
The diffusion sequence of ions is also an important factor in the study of the oxidation process of high-entropy alloys. If the diffusion rate of ions is large, the reaction rate of the ions with oxygen in the process of high-temperature oxidation will also be accelerated. If the ions with high diffusion speed react with oxygen to form a dense oxide film, the oxidation resistance of high-entropy alloys will be improved. Alternatively, if the oxide film is discontinuous after the reaction of the ions with high diffusion velocity with oxygen, or even forms relatively large voids, the oxidation resistance of the high-entropy alloy will be greatly reduced. The diffusion order of ions in FeCoNiCrMn high-entropy alloy is Mn+2 > Fe+2 > Ni+2 > Cr+3, so the diffusion rate of Mn+2 is the fastest, while the Mn3O4 formed by Mn element in the outer layer is discontinuous, leading to oxidation voids and accelerated oxidation in Mn-containing alloys [103–104]. The schematic diagram of FeCoNiCrMn oxidation is shown in Fig. 14 [45]. MnO is formed in the early stage of oxidation, and (Mn,Cr)3O4 is formed in the middle stage of oxidation. In the later stage of oxidation, (Mn,Fe)3O4 and MnO grow mixed in the outer layer.
Severe lattice distortion will introduce slow diffusion of high-entropy alloys, which will also have a certain effect on the high-temperature oxidation. Currently, serious local lattice distortion only appears in high-entropy alloys containing Zr or Hf [105–106]. According to the dislocation tube theory, when heated at 1400°C, the defects in the dislocation can promote the atom to move. The dislocation can act as a pipe and the atom can move rapidly along the pipe. During the oxidation process, there are two obvious zones in the TiZrNbTa high-entropy alloy, the TiZr-rich region and the TaNb-rich region [106]. The region containing Zr will have serious lattice distortion, resulting in obvious lattice distortion and the dislocation tube effect. The more serious the lattice distortion in the TiZr enrichment region and more dislocations, the higher the diffusion rate and oxidation rate of oxygen atoms. The uneven diffusion of oxygen atoms at 800°C creates stress concentration and cracks along the TiZr zone. When the temperature is greater than 1000°C, the oxygen atom diffuses uniformly, and the degree of lattice distortion is less than the threshold of the dislocation tube effect, which slows the diffusion rate of oxygen atoms and leads to uniform oxidation.
There is no systematic research on the mechanism of high-temperature oxidation. The research has been mainly experiments to evaluate the anti-oxidation performance. The essence of the high-temperature oxidation resistance of high-entropy alloys and nickel-based superalloys is the formation of oxide films. One of the potential advantages of alloys is the slow diffusion effect. The slow diffusion effect can reduce the combination speed between oxygen and element, increasing the activation energy of diffusion and reducing the diffusion rate, thereby improving the oxidation resistance.
The main challenges presenting high-temperature oxidation are that the upper limit temperature of high-temperature service is not high enough and there are few studies on high-temperature oxidation in complex gas environments. It is possible to explore the high-temperature oxidation behavior under ultra-high temperature (greater than 1500°C) conditions. The emergence of high-entropy alloys allows us to make new attempts in this field. Research on the complex gas environment is also of great practical significance. Our country’s aerospace industry is developing rapidly; as a result, we will need to study the oxidation behavior in the air at room temperature, as well as explore the changes in superalloys under conditions of the low oxygen content of space. Regarding the theoretical research and actual development needs of high-entropy alloys, theoretical research should start with alloying elements, lattice distortion, and slow diffusion. For the actual development needs of high-entropy alloys, the oxidation performance of high-entropy alloys can be optimized in consideration of coatings, heat treatment processes, and preparation methods.
(1) On the basis of the above analysis, alloy elements play a decisive role in the oxidation properties of high-entropy alloys. Therefore, we should start to look for alloy elements with better oxidation resistance. For example, there are a few studies on the Au element, which can enhance the properties in combination with Co, Cr, and other elements. We can use high-throughput technology to prepare different kinds of high-entropy alloys containing Au element, as well as study the effect of the proportion of Au element on the oxidation properties.
(2) Lattice distortion can affect the thermodynamics and kinetics of high-entropy alloys, which will be reflected in the high-temperature oxidation process of high-entropy alloys. The change of structure will affect the diffusion of oxygen, and even transform the diffusion path of oxygen in high-entropy alloys. When the atomic radius of metal element is large, it will lead to lattice distortion in high-entropy alloys such as Ti, Zr, and Mo. We can seek an alloying element to produce obvious lattice distortion, leading oxygen to the vicinity of Al, Cr, and other alloy elements with good oxidation resistance similar to drainage to form Al2O3 and Cr2O3, improving the oxidation resistance.
(3) The slow diffusion effect is a typical characteristic of high-entropy alloys. The diffusion rate of ions in FeCoNiCrMnis Mn+2 > Fe+2 > Ni+2 > Cr+3, which leads to Mn3O4 discontinuity formed by Mn elements in the outer layer, forming pores, and accelerating oxidation. Combining theory with experiment can be used in future research, and a potential high-entropy alloy model can be constructed through the first principle. The diffusion rates of various elements in high-entropy alloy at a high temperature can be roughly predicted, and the sequence of oxidation can be determined. Finally, experiments can be designed to identify high-entropy alloys with high-temperature oxidation resistance.
(4) Although high-entropy alloys have some price advantages, high-entropy alloy coatings on the surface of a substrate can be considered to reduce costs.
(5) Different heat treatment processes also have a great influence on the oxidation resistance of high-entropy alloys. The composition of high-entropy alloys can be more uniform through solid solution and other methods. In addition, the dense oxide film formed on the surface of the high-entropy alloy through heat treatment at a certain temperature may improve the high-temperature oxidation resistance of high-entropy alloys.
(6) Different preparation processes have different effects on high-temperature oxidation, and the preparation process has made great progress in recent years. Different preparation processes, such as casting, 3D printing, magnetron sputtering, and other processes, can be used to identify the high-entropy alloy with the best performance.
This work was financially supported by the National Natural Science Foundation of China (No. 52071014), the Fundamental Research Funds for the Central Universities (No. FRF-GF-19-033BZ), and the National Key Research and Development Program of China (No. 2020YFB0704501).
[1] |
X.B. Meng, Q. Lu, J.G. Li, T. Jin, X.F. Sun, J. Zhang, Z.Q. Chen, Y.H. Wang, and Z.Q. Hu, Modes of grain selection in spiral selector during directional solidification of nickel-base superalloys, J. Mater. Sci. Technol., 28(2012), No. 3, p. 214. DOI: 10.1016/S1005-0302(12)60044-9
|
[2] |
X.B. Meng, J.G. Li, T. Jin, X.F. Sun, C.B. Sun, and Z.Q. Hu, Evolution of grain selection in spiral selector during directional solidification of nickel-base superalloys, J. Mater. Sci. Technol., 27(2011), No. 2, p. 118. DOI: 10.1016/S1005-0302(11)60036-4
|
[3] |
R. Darolia, Development of strong, oxidation and corrosion resistant nickel-based superalloys: Critical review of challenges, progress and prospects, Int. Mater. Rev., 64(2019), No. 6, p. 355. DOI: 10.1080/09506608.2018.1516713
|
[4] |
J.H. Chen, P.M. Rogers, and J.A. Little, Oxidation behavior of several chromia-forming commercial nickel-base superalloys, Oxid. Met., 47(1997), No. 5-6, p. 381. DOI: 10.1007/BF02134783
|
[5] |
Z.H. Tan, X.G. Wang, W. Song, Y.H. Yang, J.L. Liu, J.D. Liu, L. Yang, Y.Z. Zhou, and X.F. Sun, Oxidation behavior of a novel nickel-based single crystal superalloy at elevated temperature, Vacuum, 175(2020), art. No. 109284. DOI: 10.1016/j.vacuum.2020.109284
|
[6] |
B.H. Yu, Y.P. Li, Y. Nie, and H. Mei, High temperature oxidation behavior of a novel cobalt–nickel-base superalloy, J. Alloys Compd., 765(2018), p. 1148. DOI: 10.1016/j.jallcom.2018.06.275
|
[7] |
A. Sato, Y.L. Chiu, and R.C. Reed, Oxidation of nickel-based single-crystal superalloys for industrial gas turbine applications, Acta Mater., 59(2011), No. 1, p. 225. DOI: 10.1016/j.actamat.2010.09.027
|
[8] |
B. Cantor, I.T.H. Chang, P. Knight, and A.J.B. Vincent, Microstructural development in equiatomic multicomponent alloys, Mater. Sci. Eng. A, 375-377(2004), p. 213. DOI: 10.1016/j.msea.2003.10.257
|
[9] |
J.W. Yeh, S.K. Chen, S.J. Lin, J.Y. Gan, T.S. Chin, T.T. Shun, C.H. Tsau, and S.Y. Chang, Nanostructured high-entropy alloys with multiple principal elements: Novel alloy design concepts and outcomes, Adv. Eng. Mater., 6(2004), No. 5, p. 299. DOI: 10.1002/adem.200300567
|
[10] |
D.B. Miracle, J.D. Miller, O.N. Senkov, C. Woodward, M.D. Uchic, and J. Tiley, Exploration and development of high entropy alloys for structural applications, Entropy, 16(2014), No. 1, p. 494. DOI: 10.3390/e16010494
|
[11] |
J.W. Yeh, Recent progress in high-entropy alloys, Eur. J. Control, 31(2006), No. 6, p. 633.
|
[12] |
C. Zhang, F. Zhang, K. Jin, H.B. Bei, S.L. Chen, W.S. Cao, J. Zhu, and D.C. Lv, Understanding of the elemental diffusion behavior in concentrated solid solution alloys, J. Phase Equilib. Diffus., 38(2017), No. 4, p. 434. DOI: 10.1007/s11669-017-0580-5
|
[13] |
Y. Zou, S. Maiti, W. Steurer, and R. Spolenak, Size-dependent plasticity in an Nb25Mo25Ta25W25 refractory high-entropy alloy, Acta Mater., 65(2014), p. 85. DOI: 10.1016/j.actamat.2013.11.049
|
[14] |
K.Y. Tsai, M.H. Tsai, and J.W. Yeh, Sluggish diffusion in Co–Cr–Fe–Mn–Ni high-entropy alloys, Acta Mater., 61(2013), No. 13, p. 4887. DOI: 10.1016/j.actamat.2013.04.058
|
[15] |
E.J. Pickering and N.G. Jones, High-entropy alloys: A critical assessment of their founding principles and future prospects, Int. Mater. Rev., 61(2016), No. 3, p. 183. DOI: 10.1080/09506608.2016.1180020
|
[16] |
S. Zhu, W.B. Du, X.M. Wang, and G.F. Han, High mixing entropy alloys design with high anticorrosion and wear-resistance properties, Adv. Mater. Res., 815(2013), p. 19. DOI: 10.4028/www.scientific.net/AMR.815.19
|
[17] |
J.W. Yeh, Physical metallurgy of high-entropy alloys, JOM, 67(2015), No. 10, p. 2254. DOI: 10.1007/s11837-015-1583-5
|
[18] |
Y.S. Huang, L. Chen, H.W. Lui, M.H. Cai, and J.W. Yeh, Microstructure, hardness, resistivity and thermal stability of sputtered oxide films of AlCoCrCu0.5NiFe high-entropy alloy, Mater. Sci. Eng. A, 457(2007), No. 1-2, p. 77. DOI: 10.1016/j.msea.2006.12.001
|
[19] |
S. Praveen, A. Anupam, R. Tilak, and R.S. Kottada, Phase evolution and thermal stability of AlCoCrFe high entropy alloy with carbon as unsolicited addition from milling media, Mater. Chem. Phys., 210(2018), p. 57. DOI: 10.1016/j.matchemphys.2017.10.040
|
[20] |
V. Dolique, A.L. Thomann, P. Brault, Y. Tessier, and P. Gillon, Thermal stability of AlCoCrCuFeNi high entropy alloy thin films studied by in-situ XRD analysis, Surf. Coat. Technol., 204(2010), No. 12-13, p. 1989. DOI: 10.1016/j.surfcoat.2009.12.006
|
[21] |
A. Karati, K. Guruvidyathri, V.S. Hariharan, and B.S. Murty, Thermal stability of AlCoFeMnNi high-entropy alloy, Scripta Mater., 162(2019), p. 465. DOI: 10.1016/j.scriptamat.2018.12.017
|
[22] |
Q.L. Xu, Y. Zhang, S.H. Liu, C.J. Li, and C.X. Li, High-temperature oxidation behavior of CuAlNiCrFe high-entropy alloy bond coats deposited using high-speed laser cladding process, Surf. Coat. Technol., 398(2020), art. No. 126093. DOI: 10.1016/j.surfcoat.2020.126093
|
[23] |
Y.P. Lin, T.F. Yang, L. Lang, C. Shan, H.Q. Deng, W.Y. Hu, and F. Gao, Enhanced radiation tolerance of the Ni–Co–Cr–Fe high-entropy alloy as revealed from primary damage, Acta Mater., 196(2020), p. 133. DOI: 10.1016/j.actamat.2020.06.027
|
[24] |
C.M. Barr, J.E. Nathaniel, K.A. Unocic, J.P. Liu, Y. Zhang, Y.Q. Wang, and M.L. Taheri, Exploring radiation induced segregation mechanisms at grain boundaries in equiatomic CoCrFeNiMn high entropy alloy under heavy ion irradiation, Scripta Mater., 156(2018), p. 80. DOI: 10.1016/j.scriptamat.2018.06.041
|
[25] |
G. Pu, L.W. Lin, R. Ang, K. Zhang, B. Liu, B. Liu, T. Peng, S.F. Liu, and Q.R. Li, Outstanding radiation tolerance and mechanical behavior in ultra-fine nanocrystalline Al1.5CoCrFeNi high entropy alloy films under He ion irradiation, Appl. Surf. Sci., 516(2020), art. No. 146129. DOI: 10.1016/j.apsusc.2020.146129
|
[26] |
D. Patel, M.D. Richardson, B. Jim, S. Akhmadaliev, R. Goodall, and A.S. Gandy, Radiation damage tolerance of a novel metastable refractory high entropy alloy V2.5Cr1.2WMoCo0.04, J. Nucl. Mater., 531(2020), art. No. 152005. DOI: 10.1016/j.jnucmat.2020.152005
|
[27] |
P. Lu, J.E. Saal, G.B. Olson, T.S. Li, O.J. Swanson, G.S. Frankel, A.Y. Gerard, K.F. Quiambao, and J.R. Scully, Computational materials design of a corrosion resistant high entropy alloy for harsh environments, Scripta Mater., 153(2018), p. 19. DOI: 10.1016/j.scriptamat.2018.04.040
|
[28] |
S. Shuang, Z.Y. Ding, D. Chung, S.Q. Shi, and Y. Yang, Corrosion resistant nanostructured eutectic high entropy alloy, Corros. Sci., 164(2020), art. No. 108315. DOI: 10.1016/j.corsci.2019.108315
|
[29] |
P. Muangtong, A. Rodchanarowan, D. Chaysuwan, N. Chanlek, and R. Goodall, The corrosion behaviour of CoCrFeNi–x (x = Cu, Al, Sn) high entropy alloy systems in chloride solution, Corros. Sci., 172(2020), art. No. 108740. DOI: 10.1016/j.corsci.2020.108740
|
[30] |
X. Wang and Y.P. Zhang, Microstructures and corrosion resistance properties of as-cast and homogenized AlFeNiCuCr high entropy alloy, Mater. Chem. Phys., 254(2020), art. No. 123440. DOI: 10.1016/j.matchemphys.2020.123440
|
[31] |
X.Y. Wang, Q. Liu, Y.B. Huang, L. Xie, Q. Xu, and T.X. Zhao, Effect of Ti content on the microstructure and corrosion resistance of CoCrFeNiTix high entropy alloys prepared by laser cladding, Materials, 13(2020), No. 10, art. No. 2209. DOI: 10.3390/ma13102209
|
[32] |
H. Cheng, Y.C. Lin, D.G. He, Y.L. Qiu, J.C. Zhu, and M.S. Chen, Influences of stress-aging on the precipitation behavior of δ phase (Ni3Nb) in a nickel-based superalloy, Mater. Des., 197(2021), art. No. 109256. DOI: 10.1016/j.matdes.2020.109256
|
[33] |
J. Yang, J. Wu, C.Y. Zhang, S.D. Zhang, B.J. Yang, W. Emori, and J.Q. Wang, Effects of Mn on the electrochemical corrosion and passivation behavior of CoFeNiMnCr high-entropy alloy system in H2SO4 solution, J. Alloys Compd., 819(2020), art. No. 152943. DOI: 10.1016/j.jallcom.2019.152943
|
[34] |
R.B. Nair, H.S. Arora, and H.S. Grewal, Enhanced cavitation erosion resistance of a friction stir processed high entropy alloy, Int. J. Miner. Metall. Mater., 27(2020), No. 10, p. 1353. DOI: 10.1007/s12613-020-2000-9
|
[35] |
N. Malatji, A.P.I. Popoola, T. Lengopeng, and S. Pityana, Effect of Nb addition on the microstructural, mechanical and electrochemical characteristics of AlCrFeNiCu high-entropy alloy, Int. J. Miner. Metall. Mater., 27(2020), No. 10, p. 1332. DOI: 10.1007/s12613-020-2178-x
|
[36] |
H. Jiang, D.X. Qiao, W.N. Jiao, K.M. Han, Y.P. Lu, and P.K. Liaw, Tensile deformation behavior and mechanical properties of a bulk cast Al0.9CoFeNi2 eutectic high-entropy alloy, J. Mater. Sci. Technol., 61(2021), p. 119. DOI: 10.1016/j.jmst.2020.05.053
|
[37] |
W.Y. Zhang, D.S. Yan, W.J. Lu, and Z.M. Li, Carbon and nitrogen co-doping enhances phase stability and mechanical properties of a metastable high-entropy alloy, J. Alloys Compd., 831(2020), art. No. 154799. DOI: 10.1016/j.jallcom.2020.154799
|
[38] |
H. Ma and C.H. Shek, Effects of Hf on the microstructure and mechanical properties of CoCrFeNi high entropy alloy, J. Alloys Compd., 827(2020), art. No. 154159. DOI: 10.1016/j.jallcom.2020.154159
|
[39] |
Y. Dong, Z.Q. Yao, X. Huang, F.M. Du, C.Q. Li, A.F. Chen, F. Wu, Y.Q. Cheng, and Z.R. Zhang, Microstructure and mechanical properties of AlCoxCrFeNi3−x eutectic high-entropy-alloy system, J. Alloys Compd., 823(2020), art. No. 153886. DOI: 10.1016/j.jallcom.2020.153886
|
[40] |
Q.Q. Wei, G.Q. Luo, J. Zhang, P.G. Chen, Q. Shen, and L.M. Zhang, Effect of raw material forms on the microstructure and mechanical properties of MoNbRe0.5TaW high-entropy alloy, Mater. Sci. Eng. A, 794(2020), art. No. 139632. DOI: 10.1016/j.msea.2020.139632
|
[41] |
Z. Wang, C. Wang, Y.L. Zhao, T.H. Huang, C.L. Li, J.J. Kai, C.T. Liu, and C.H. Hsueh, Growth, microstructure and mechanical properties of CoCrFeMnNi high entropy alloy films, Vacuum, 179(2020), art. No. 109553. DOI: 10.1016/j.vacuum.2020.109553
|
[42] |
M. Zhang, J.X. Hou, H.J. Yang, Y.Q. Tan, X.J. Wang, X.H. Shi, R.P. Guo, and J.W. Qiao, Tensile strength prediction of dual-phase Al0.6CoCrFeNi high-entropy alloys, Int. J. Miner. Metall. Mater., 27(2020), No. 10, p. 1341. DOI: 10.1007/s12613-020-2084-2
|
[43] |
M. Kang, K.R. Lim, J.W. Won, K.S. Lee, and Y.S. Na, Al–Ti-containing lightweight high-entropy alloys for intermediate temperature applications, Entropy, 20(2018), No. 5, art. No. 355. DOI: 10.3390/e20050355
|
[44] |
W. Kai, F.C. Chien, F.P. Cheng, R.T. Huang, J.J. Kai, and C.T. Liu, The corrosion of an equimolar FeCoNiCrMn high-entropy alloy in various CO2/CO mixed gases at 700 and 950°C, Corros. Sci., 153(2019), p. 150. DOI: 10.1016/j.corsci.2019.03.030
|
[45] |
W. Kai, F.P. Cheng, F.C. Chien, Y.R. Lin, D. Chen, J.J. Kai, C.T. Liu, and C.J. Wang, The oxidation behavior of a Ni2FeCoCrAl0.5 high-entropy superalloy in O2-containing environments, Corros. Sci., 158(2019), art. No. 108093. DOI: 10.1016/j.corsci.2019.108093
|
[46] |
H. Shi, C.C. Tang, A. Jianu, R. Fetzer, A. Weisenburger, M. Steinbrueck, M. Grosse, R. Stieglitz, and G. Müller, Oxidation behavior and microstructure evolution of alumina-forming austenitic & high entropy alloys in steam environment at 1200°C, Corros. Sci., 170(2020), art. No. 108654. DOI: 10.1016/j.corsci.2020.108654
|
[47] |
W. Kai, F.P. Cheng, C.Y. Liao, C.C. Li, R.T. Huang, and J.J. Kai, The oxidation behavior of the quinary FeCoNiCrSix high-entropy alloys, Mater. Chem. Phys., 210(2018), p. 362. DOI: 10.1016/j.matchemphys.2017.06.017
|
[48] |
W. Kai, F.P. Cheng, Y.R. Lin, C.W. Chuang, R.T. Huang, D. Chen, J.J. Kai, C.T. Liu, and C.J. Wang, The oxidation behavior of Ni2FeCoCrAlx high-entropy alloys in dry air, J. Alloys Compd., 836(2020), art. No. 155518. DOI: 10.1016/j.jallcom.2020.155518
|
[49] |
D. Huang, J.S. Lu, Y.X. Zhuang, C.X. Tian, and Y.B. Li, The role of Nb on the high temperature oxidation behavior of CoCrFeMnNbxNi high-entropy alloys, Corros. Sci., 158(2019), art. No. 108088. DOI: 10.1016/j.corsci.2019.07.012
|
[50] |
X. Yang and Y. Zhang, Prediction of high-entropy stabilized solid-solution in multi-component alloys, Mater. Chem. Phys., 132(2012), No. 2-3, p. 233. DOI: 10.1016/j.matchemphys.2011.11.021
|
[51] |
B. Gorr, M. Azim, H.J. Christ, T. Mueller, D. Schliephake, and M. Heilmaier, Phase equilibria, microstructure, and high temperature oxidation resistance of novel refractory high-entropy alloys, J. Alloys Compd., 624(2015), p. 270. DOI: 10.1016/j.jallcom.2014.11.012
|
[52] |
V. Pacheco, G. Lindwall, D. Karlsson, J. Cedervall, S. Fritze, G. Ek, P. Berastegui, M. Sahlberg, and U. Jansson, Thermal stability of the HfNbTiVZr high-entropy alloy, Inorg. Chem., 58(2019), No. 1, p. 811. DOI: 10.1021/acs.inorgchem.8b02957
|
[53] |
C.V.S. Raju, D. Venugopal, P.R. Srikanth, K. Lokeshwaran, M. Srinivas, C.J. Chary, and A.A. Kumar, Effect of aluminum addition on the properties of CoCuFeNiTi high entropy alloys, Mater. Today: Proc., 5(2018), No. 13, p. 26823. DOI: 10.1016/j.matpr.2018.08.163
|
[54] |
C.W. Tsai, M.H. Tsai, J.W. Yeh, and C.C. Yang, Effect of temperature on mechanical properties of Al0.5CoCrCuFeNi wrought alloy, J. Alloys Compd., 490(2010), No. 1-2, p. 160. DOI: 10.1016/j.jallcom.2009.10.088
|
[55] |
S.F. Ge, H.M. Fu, L. Zhang, H.H. Mao, H. Li, A.M. Wang, W.R. Li, and H.F. Zhang, Effects of Al addition on the microstructures and properties of MoNbTaTiV refractory high entropy alloy, Mater. Sci. Eng. A, 784(2020), art. No. 139275. DOI: 10.1016/j.msea.2020.139275
|
[56] |
Y.Y. Liu, Z. Chen, J.C. Shi, Z.Y. Wang, and J.Y. Zhang, The effect of Al content on microstructures and comprehensive properties in AlxCoCrCuFeNi high entropy alloys, Vacuum, 161(2019), p. 143. DOI: 10.1016/j.vacuum.2018.12.009
|
[57] |
Y. Cui, J.Q. Shen, S.M. Manladan, K.P. Geng, and S.S. Hu, Wear resistance of FeCoCrNiMnAlx high-entropy alloy coatings at high temperature, Appl. Surf. Sci., 512(2020), art. No. 145736. DOI: 10.1016/j.apsusc.2020.145736
|
[58] |
S.A. Uporov, R.E. Ryltsev, V.A. Bykov, S.K. Estemirova, and D.A. Zamyatin, Microstructure, phase formation and physical properties of AlCoCrFeNiMn high-entropy alloy, J. Alloys Compd., 820(2020), art. No. 153228. DOI: 10.1016/j.jallcom.2019.153228
|
[59] |
J. Lu, Y. Chen, H. Zhang, L.M. He, R.D. Mu, Z.Y. Shen, X.F. Zhao, and F.W. Guo, Y/Hf-doped Al0.7CoCrFeNi high-entropy alloy with ultra oxidation and spallation resistance at 1200°C, Corros. Sci., 174(2020), art. No. 108803. DOI: 10.1016/j.corsci.2020.108803
|
[60] |
J. Lu, Y. Chen, H. Zhang, L. Li, L.M. Fu, X.F. Zhao, F.W. Guo, and P. Xiao, Effect of Al content on the oxidation behavior of Y/Hf-doped AlCoCrFeNi high-entropy alloy, Corros. Sci., 170(2020), art. No. 108691. DOI: 10.1016/j.corsci.2020.108691
|
[61] |
Z.Y. Rao, X. Wang, Q.J. Wang, T. Liu, X.H. Chen, L. Wang, and X.D. Hui, Microstructure, mechanical properties, and oxidation behavior of AlxCr0.4CuFe0.4MnNi high entropy alloys, Adv. Eng. Mater., 19(2017), No. 5, art. No. 1600726. DOI: 10.1002/adem.201600726
|
[62] |
Y.C. Cai, L.S. Zhu, Y. Cui, K.P. Geng, S.M. Manladan, and Z. Luo, High-temperature oxidation behavior of FeCoCrNiAlx high-entropy alloy coatings, Mater. Res. Express, 6(2019), No. 12, art. No. 126552. DOI: 10.1088/2053-1591/ab562d
|
[63] |
M.V. Karpets, V.F. Gorban, O.A. Rokitska, M.O. Krapivka, E.S. Makarenko, and A.V. Samelyuk, Features of high-temperature oxidation of high-entropy AlCrFe3CoNiCu alloy, Powder Metall. Met. Ceram., 57(2018), No. 3-4, p. 221. DOI: 10.1007/s11106-018-9972-2
|
[64] |
S. Guo, C. Ng, J. Lu, and C.T. Liu, Effect of valence electron concentration on stability of fcc or bcc phase in high entropy alloys, J. Appl. Phys., 109(2011), No. 10, art. No. 103505. DOI: 10.1063/1.3587228
|
[65] |
T.M. Butler and M.L. Weaver, Oxidation behavior of arc melted AlCoCrFeNi multi-component high-entropy alloys, J. Alloys Compd., 674(2016), p. 229. DOI: 10.1016/j.jallcom.2016.02.257
|
[66] |
Y.Y. Liu, Z. Chen, Y.Z. Chen, J.C. Shi, Z.Y. Wang, S. Wang, and F. Liu, Effect of Al content on high temperature oxidation resistance of AlxCoCrCuFeNi high entropy alloys (x=0, 0.5, 1, 1.5, 2), Vacuum, 169(2019), art. No. 108837. DOI: 10.1016/j.vacuum.2019.108837
|
[67] |
N.Y. Bao, J. Zuo, Z.Y. Du, M.L. Yang, G. Jiang, and L. Zhang, Computational characterization of the structural and mechanical properties of AlxCoCrFeNiTi1−x high entropy alloys, Mater. Res. Express, 6(2019), No. 9, art. No. 096519. DOI: 10.1088/2053-1591/ab2b77
|
[68] |
A. Mohanty, J.K. Sampreeth, O. Bembalge, J.Y. Hascoet, S. Marya, R.J. Immanuel, and S.K. Panigrahi, High temperature oxidation study of direct laser deposited AlXCoCrFeNi (X=0.3,0.7) high entropy alloys, Surf. Coat. Technol., 380(2019), art. No. 125028. DOI: 10.1016/j.surfcoat.2019.125028
|
[69] |
F.X. Ye, Z.P. Jiao, S. Yan, L. Guo, L.Z. Feng, and J.X. Yu, Microbeam plasma arc remanufacturing: Effects of Al on microstructure, wear resistance, corrosion resistance and high temperature oxidation resistance of AlxCoCrFeMnNi high-entropy alloy cladding layer, Vacuum, 174(2020), art. No. 109178. DOI: 10.1016/j.vacuum.2020.109178
|
[70] |
L.C. Li, M.X. Li, M. Liu, B.Y. Sun, C. Wang, J.T. Huo, W.H. Wang, and Y.H. Liu, Enhanced oxidation resistance of MoTaTiCrAl high entropy alloys by removal of Al, Sci. China Mater., 64(2021), No. 1, p. 223. DOI: 10.1007/s40843-020-1332-2
|
[71] |
A. Erdogan, K.M. Doleker, and S. Zeytin, Effect of Al and Ti on high-temperature oxidation behavior of CoCrFeNi-based high-entropy alloys, JOM, 71(2019), No. 10, p. 3499. DOI: 10.1007/s11837-019-03679-2
|
[72] |
G.S. Ham, Y.K. Kim, Y.S. Na, and K.A. Lee, Effect of Ti addition on the microstructure and high-temperature oxidation property of AlCoCrFeNi high-entropy alloy, Met. Mater. Int., 27(2021), No. 1, p. 156. DOI: 10.1007/s12540-020-00708-7
|
[73] |
Q.D. Qin, J.B. Qu, Y.E. Hu, Y.J. Wu, and X.D. Su, Microstructural characterization and oxidation resistance of multicomponent equiatomic CoCrCuFeNi–TiO high-entropy alloy, Int. J. Miner. Metall. Mater., 25(2018), No. 11, p. 1286. DOI: 10.1007/s12613-018-1681-9
|
[74] |
J. Dąbrowa, G. Cieślak, M. Stygar, K. Mroczka, K. Berent, T. Kulik, and M. Danielewski, Influence of Cu content on high temperature oxidation behavior of AlCoCrCuxFeNi high entropy alloys (x=0; 0.5; 1), Intermetallics, 84(2017), p. 52. DOI: 10.1016/j.intermet.2016.12.015
|
[75] |
F. Chang, B.J. Cai, C. Zhang, B. Huang, S. Li, and P.Q. Dai, Thermal stability and oxidation resistance of FeCrxCoNiB high-entropy alloys coatings by laser cladding, Surf. Coat. Technol., 359(2019), p. 132. DOI: 10.1016/j.surfcoat.2018.12.072
|
[76] |
Y.J. Chang and A.C. Yeh, The evolution of microstructures and high temperature properties of AlxCo1.5CrFeNi1.5Tiy high entropy alloys, J. Alloys Compd., 653(2015), p. 379. DOI: 10.1016/j.jallcom.2015.09.042
|
[77] |
W. Kai, C.C. Li, F.P. Cheng, K.P. Chu, R.T. Huang, L.W. Tsay, and J.J. Kai, Air-oxidation of FeCoNiCr-based quinary high-entropy alloys at 700–900°C, Corros. Sci., 121(2017), p. 116. DOI: 10.1016/j.corsci.2017.02.008
|
[78] |
G. Laplanche, U.F. Volkert, G. Eggeler, and E.P. George, Oxidation behavior of the CrMnFeCoNi high-entropy alloy, Oxid. Met., 85(2016), No. 5-6, p. 629. DOI: 10.1007/s11085-016-9616-1
|
[79] |
B. Gorr, F. Mueller, H.J. Christ, T. Mueller, H. Chen, A. Kauffmann, and M. Heilmaier, High temperature oxidation behavior of an equimolar refractory metal-based alloy 20Nb–20Mo–20Cr–20Ti–20Al with and without Si addition, J. Alloys Compd., 688(2016), p. 468.
|
[80] |
S. Shajahan, A. Kumar, M. Chopkar, and A. Basu, Oxidation study of CoCrCuFeNiSix high entropy alloys, Mater. Res. Express, 7(2020), No. 1, art. No. 016532. DOI: 10.1088/2053-1591/ab640a
|
[81] |
F. Müller, B. Gorr, H.J. Christ, H. Chen, A. Kauffmann, and M. Heilmaier, Effect of microalloying with silicon on high temperature oxidation resistance of novel refractory high-entropy alloy Ta–Mo–Cr–Ti–Al, Mater. High Temp., 35(2018), No. 1-3, p. 168. DOI: 10.1080/09603409.2017.1389115
|
[82] |
S. Wang, Y. Wu, C.S. Ni, and Y. Niu, The effect of Si additions on the high temperature oxidation of a ternary Ni–10Cr–4Al alloy in 1 atm O2 at 1100°C, Corros. Sci., 51(2009), No. 3, p. 511. DOI: 10.1016/j.corsci.2008.10.023
|
[83] |
J.J. Yang, C.M. Kuo, P.T. Lin, H.C. Liu, C.Y. Huang, H.W. Yen, and C.W. Tsai, Improvement in oxidation behavior of Al0.2Co1.5CrFeNi1.5Ti0.3 high-entropy superalloys by minor Nb addition, J. Alloys Compd., 825(2020), art. No. 153983. DOI: 10.1016/j.jallcom.2020.153983
|
[84] |
S. Sheikh, M.K. Bijaksana, A. Motallebzadeh, S. Shafeie, A. Lozinko, L. Gan, T.K. Tsao, U. Klement, D. Canadinc, H. Murakami, and S. Guo, Accelerated oxidation in ductile refractory high-entropy alloys, Intermetallics, 97(2018), p. 58. DOI: 10.1016/j.intermet.2018.04.001
|
[85] |
Y.K. Cao, Y. Liu, B. Liu, W.D. Zhang, J.W. Wang, and M. Du, Effects of Al and Mo on high temperature oxidation behavior of refractory high entropy alloys, Trans. Nonferrous Met. Soc. China, 29(2019), No. 7, p. 1476. DOI: 10.1016/S1003-6326(19)65054-5
|
[86] |
O.A. Waseem and H.J. Ryu, Combinatorial synthesis and analysis of AlxTayVz–Cr20Mo20Nb20 Ti20Zr10 and Al10CrMoxNbTiZr10 refractory high-entropy alloys: Oxidation behavior, J. Alloys Compd., 828(2020), art. No. 154427. DOI: 10.1016/j.jallcom.2020.154427
|
[87] |
B. Gorr, F. Müller, S. Schellert, H.J. Christ, H. Chen, A. Kauffmann, and M. Heilmaier, A new strategy to intrinsically protect refractory metal based alloys at ultra high temperatures, Corros. Sci., 166(2020), art. No. 108475. DOI: 10.1016/j.corsci.2020.108475
|
[88] |
F. Müller, B. Gorr, H.J. Christ, J. Müller, B. Butz, H. Chen, A. Kauffmann, and M. Heilmaier, On the oxidation mechanism of refractory high entropy alloys, Corros. Sci., 159(2019), art. No. 108161. DOI: 10.1016/j.corsci.2019.108161
|
[89] |
K.C. Lo, Y.J. Chang, H. Murakami, J.W. Yeh, and A.C. Yeh, An oxidation resistant refractory high entropy alloy protected by CrTaO4-based oxide, Sci. Rep., 9(2019), art. No. 7266. DOI: 10.1038/s41598-019-43819-x
|
[90] |
Y.X. Guo, H.L. Wang, and Q.B. Liu, Microstructure evolution and strengthening mechanism of laser-cladding MoFexCrTiWAlNby refractory high-entropy alloy coatings, J. Alloys Compd., 834(2020), art. No. 155147. DOI: 10.1016/j.jallcom.2020.155147
|
[91] |
L.L. Hou, J.T. Hui, Y.H. Yao, J. Chen, and J.N. Liu, Effects of boron content on microstructure and mechanical properties of AlFeCoNiBx high entropy alloy prepared by vacuum arc melting, Vacuum, 164(2019), p. 212. DOI: 10.1016/j.vacuum.2019.03.019
|
[92] |
V. Shivam, Y. Shadangi, J. Basu, and N.K. Mukhopadhyay, Evolution of phases, hardness and magnetic properties of AlCoCrFeNi high entropy alloy processed by mechanical alloying, J. Alloys Compd., 832(2020), art. No. 154826. DOI: 10.1016/j.jallcom.2020.154826
|
[93] |
D. Oleszak, A. Antolak-Dudka, and T. Kulik, High entropy multicomponent WMoNbZrV alloy processed by mechanical alloying, Mater. Lett., 232(2018), p. 160. DOI: 10.1016/j.matlet.2018.08.060
|
[94] |
H. Gedda, A. Kaplan, and J. Powell, Melt-solid interactions in laser cladding and laser casting, Metall. Mater. Trans. B, 36(2005), No. 5, p. 683. DOI: 10.1007/s11663-005-0059-3
|
[95] |
C.H. Lai, S.J. Lin, J.W. Yeh, and S.Y. Chang, Preparation and characterization of AlCrTaTiZr multi-element nitride coatings, Surf. Coat. Technol., 201(2006), No. 6, p. 3275. DOI: 10.1016/j.surfcoat.2006.06.048
|
[96] |
Y.Z. Shi, B. Yang, P.D. Rack, S.F. Guo, P.K. Liaw, and Y. Zhao, High-throughput synthesis and corrosion behavior of sputter-deposited nanocrystalline Alx(CoCrFeNi)100−x combinatorial high-entropy alloys, Mater. Des., 195(2020), art. No. 109018. DOI: 10.1016/j.matdes.2020.109018
|
[97] |
S. Shukla, T.H. Wang, M. Frank, P. Agrawal, S. Sinha, R.A. Mirshams, and R.S. Mishra, Friction stir gradient alloying: A novel solid-state high throughput screening technique for high entropy alloys, Mater. Today Commun., 23(2020), art. No. 100869. DOI: 10.1016/j.mtcomm.2019.100869
|
[98] |
Y.Q. Xu, Y.Q. Bu, J.B. Liu, and H.T. Wang, In-situ high throughput synthesis of high-entropy alloys, Scripta Mater., 160(2019), p. 44. DOI: 10.1016/j.scriptamat.2018.09.040
|
[99] |
M. Li, J. Gazquez, A. Borisevich, R. Mishra, and K.M. Flores, Evaluation of microstructure and mechanical property variations in AlxCoCrFeNi high entropy alloys produced by a high-throughput laser deposition method, Intermetallics, 95(2018), p. 110. DOI: 10.1016/j.intermet.2018.01.021
|
[100] |
X.Y. Gao and Y.Z. Lu, Laser 3D printing of CoCrFeMnNi high-entropy alloy, Mater. Lett., 236(2019), p. 77. DOI: 10.1016/j.matlet.2018.10.084
|
[101] |
Y. Hong, M.B. Kivy, and M.A. Zaeem, Competition between formation of Al2O3 and Cr2O3 in oxidation of Al0.3CoCrCuFeNi high entropy alloy: A first-principles study, Scripta Mater., 168(2019), p. 139. DOI: 10.1016/j.scriptamat.2019.04.041
|
[102] |
I. Barin, Thermochemical Data for Pure Substances, 3rd ed., VCH Verlagsgesellschaft mbH, Weinheim, 1995.
|
[103] |
W. Kai, C.C. Li, F.P. Cheng, K.P. Chu, R.T. Huang, L.W. Tsay, and J.J. Kai, The oxidation behavior of an equimolar FeCoNiCrMn high-entropy alloy at 950°C in various oxygen-containing atmospheres, Corros. Sci., 108(2016), p. 209. DOI: 10.1016/j.corsci.2016.03.020
|
[104] |
R.E. Lobnig, H.P. Schmidt, K. Hennesen, and H.J. Grabke, Diffusion of cations in chromia layers grown on iron-base alloys, Oxid. Met., 37(1992), No. 1-2, p. 81. DOI: 10.1007/BF00665632
|
[105] |
R.X. Wang, Y. Tang, S. Li, Y.L. Ai, Y.Y. Li, B. Xiao, L.A. Zhu, X.Y. Liu, and S.X. Bai, Effect of lattice distortion on the diffusion behavior of high-entropy alloys, J. Alloys Compd., 825(2020), art. No. 154099. DOI: 10.1016/j.jallcom.2020.154099
|
[106] |
Y. Tong, S.J. Zhao, H.B. Bei, T. Egami, Y.W. Zhang, and F.X. Zhang, Severe local lattice distortion in Zr- and/or Hf-containing refractory multi-principal element alloys, Acta Mater., 183(2020), p. 172. DOI: 10.1016/j.actamat.2019.11.026
|
[1] | Jishuo Han, Yong Li, Chenhong Ma, Qingyao Zheng, Xiuhua Zhang, Xiaofang Wu. Study on the oxidation mechanism of Al–SiC composite at elevated temperature [J]. International Journal of Minerals, Metallurgy and Materials, 2024, 31(9): 2077-2087. DOI: 10.1007/s12613-023-2778-3 |
[2] | C. D. Gómez-Esparza, A. Duarte-Moller, C. López-Díaz de León, R. Martínez-Sánchez, J. F. Hernández-Paz, C. A. Rodríguez-González. Influence of ZnO nanoparticles on the microstructure of a CoCrFeMoNi matrix via powder metallurgy [J]. International Journal of Minerals, Metallurgy and Materials, 2019, 26(11): 1467-1476. DOI: 10.1007/s12613-019-1863-0 |
[3] | Qing-dong Qin, Jin-bo Qu, Yong-e Hu, Yu-jiao Wu, Xiang-dong Su. Microstructural characterization and oxidation resistance of multicomponent equiatomic CoCrCuFeNi-TiO high-entropy alloy [J]. International Journal of Minerals, Metallurgy and Materials, 2018, 25(11): 1286-1293. DOI: 10.1007/s12613-018-1681-9 |
[4] | Xiang Li, Yan-ping Bao, Min Wang, Lu Lin. Simulation study on factors influencing the entrainment behavior of liquid steel as bubbles pass through the steel/slag interface [J]. International Journal of Minerals, Metallurgy and Materials, 2016, 23(5): 511-519. DOI: 10.1007/s12613-016-1262-8 |
[5] | İ. Afşin Kariper. Hardness of Mn2V2O7 thin films and its influential factors [J]. International Journal of Minerals, Metallurgy and Materials, 2015, 22(9): 987-991. DOI: 10.1007/s12613-015-1159-y |
[6] | Guo-liang Zhang, Sheng-li Wu, Bo Su, Zhi-gang Que, Chao-gang Hou, Yao Jiang. Influencing factor of sinter body strength and its effects on iron ore sintering indexes [J]. International Journal of Minerals, Metallurgy and Materials, 2015, 22(6): 553-561. DOI: 10.1007/s12613-015-1107-x |
[7] | Yan-ping Zeng, Peng-yu Zhu, Ke Tong. Effect of microstructure on the low temperature toughness of high strength pipeline steels [J]. International Journal of Minerals, Metallurgy and Materials, 2015, 22(3): 254-261. DOI: 10.1007/s12613-015-1069-z |
[8] | Guo-liang Zhang, Sheng-li Wu, Juan Zhu, Yong-zhi Wang. Influencing factors and mechanism of water absorption process of iron ores during sintering [J]. International Journal of Minerals, Metallurgy and Materials, 2014, 21(2): 122-130. DOI: 10.1007/s12613-014-0874-0 |
[9] | Meifeng Cai, Lan Qiao, Bo Yu, Changhong Li. Field Investigation and FEM Analysis of Ground Subsidence in a Chinese Underground Gold Mine [J]. International Journal of Minerals, Metallurgy and Materials, 2000, 7(1): 1-4. |
[10] | LIU Haiping, QI Huibin, HAO Chanshan, WANG Xiaohong, HE Yedong, ZHU Rizhang. Factors Influencing the Sulfidation Rate of Fe-Mo Binary Alloys [J]. International Journal of Minerals, Metallurgy and Materials, 1997, 4(4): 39-42. |
1. | C.B. Li, Y. Zhang, Z.Q. Wang, et al. Experimental investigation and thermodynamic re-assessment of the Fe-Ni-W phase diagram. Calphad, 2025, 88: 102793. DOI:10.1016/j.calphad.2024.102793 |
2. | Yanlong Wang, Buyu Dang, Xudong Zhang, et al. Preparation and Oxidation Resistance of CrFeCoNiCu High-Entropy Alloy Coating affected by Substrate Temperature. Journal of Materials Engineering and Performance, 2025. DOI:10.1007/s11665-025-10997-x |
3. | Jian Zhu, Kefeng Lu, Xidong Hui, et al. Roles of Al on microstructures, mechanical properties and oxidation resistances of FCC Al CoCrNiFe high entropy alloy coatings prepared by laser directed energy deposition. Materials Science and Engineering: A, 2025, 922: 147626. DOI:10.1016/j.msea.2024.147626 |
4. | Zengyuan Fan, Jiawei Wang, Yunpeng Wu, et al. Advanced high-entropy materials for high-quality energy storage and conversion. Energy Storage Materials, 2025, 74: 103954. DOI:10.1016/j.ensm.2024.103954 |
5. | Min Guo, Chaoyang Chen, Bin Song, et al. Effect of Al on the Oxidation Behavior of TiCrZrNbTa High-Entropy Coatings on Zr Alloy. Materials, 2025, 18(9): 1997. DOI:10.3390/ma18091997 |
6. | Heng Tao, Zhaohui Wang, Xuming Wu, et al. Effect of Al content on high-temperature oxidation behavior and mechanism of AlxCoCrFeNi1.5Ti0.1 high-entropy alloy coatings by laser cladding. Surface and Coatings Technology, 2025, 502: 131961. DOI:10.1016/j.surfcoat.2025.131961 |
7. | Maolong Chen, Xuefeng Yang, Zhiqiang Zhang, et al. Research status of laser cladding technology on aluminum alloy surface. The International Journal of Advanced Manufacturing Technology, 2025, 137(1-2): 1. DOI:10.1007/s00170-025-15204-8 |
8. | Zhenyu Wang, Ying Lou, Weimeng Zong, et al. High temperature oxidation behavior of laser-cladded refractory (Nb36Ni36Mo3Cr19Si3Zr3)100-xAlx high-entropy coatings. Materials Today Communications, 2025, 42: 111498. DOI:10.1016/j.mtcomm.2025.111498 |
9. | Bo Yuan, Harry Hey, Christopher M. Harvey, et al. Nonuniform creep-induced alumina scale spallation on FeCrAl coatings. International Journal of Mechanical Sciences, 2025, 293: 110168. DOI:10.1016/j.ijmecsci.2025.110168 |
10. | Bowen Tang, Zhichao Shang, Weijia Guo, et al. Effect of Al variation on microstructure and properties of porous FeCoNiCrAlx high-entropy alloys synthesized via thermal explosion. Intermetallics, 2025, 180: 108687. DOI:10.1016/j.intermet.2025.108687 |
11. | Fan Yang, Chao Du, Siyuan Tao, et al. Study on the effect and growth mechanism of micro-arc oxidation coating on AlCoCrFeNi high entropy alloy. Journal of Alloys and Compounds, 2025, 1020: 179469. DOI:10.1016/j.jallcom.2025.179469 |
12. | Long Zhou, Chun Li, Chenghao Zhang, et al. The outstanding high-temperature oxidation resistance of SiC joint achieved via ultrafast high-temperature joining using FeCoCrNiCu high entropy alloy. Ceramics International, 2025. DOI:10.1016/j.ceramint.2025.03.222 |
13. | Alireza Nazarahari, Huseyin Can Ozdemir, Khemais Barienti, et al. On the Surface Property–Oxidation Relationship in Refractory High‐Entropy Alloys. Advanced Engineering Materials, 2024, 26(23) DOI:10.1002/adem.202400960 |
14. | Qin Xu, Chengyuan Guo, Qi Wang, et al. Formation of Laves Phase and Its Effect on Microstructure and Mechanical Properties of Ti8Zr6Nb4V5Crx Lightweight High‐Entropy Alloys. steel research international, 2024. DOI:10.1002/srin.202400700 |
15. | Hao LI, Shengqiang YANG, Xiuhong LI, et al. Failure mechanisms and surface treatment processes of thermal barrier coatings: Review. Chinese Journal of Aeronautics, 2024, 37(9): 20. DOI:10.1016/j.cja.2024.07.015 |
16. | Haofei Sun, Jing Liu. Hot corrosion of Fe-Cr-Ni multi-principal element alloys in Na2SO4+25%NaCl mixture salts at 700–900°C. Intermetallics, 2024, 166: 108203. DOI:10.1016/j.intermet.2024.108203 |
17. | Yuntian Lou, Weiwei Chang, Yu Zhang, et al. Microbiologically influenced corrosion resistance enhancement of copper-containing high entropy alloy FexCu(1−x)CoNiCrMn against Pseudomonas aeruginosa. International Journal of Minerals, Metallurgy and Materials, 2024, 31(11): 2488. DOI:10.1007/s12613-024-2932-6 |
18. | Anne Bastin, Taylor Robertson, Xiao Huang, et al. Long‐Term High‐Temperature Oxidation of Al10CoCrFeNi30 High‐Entropy Alloy. Advanced Engineering Materials, 2024, 26(20) DOI:10.1002/adem.202400650 |
19. | Deepsovan Mondal, Jayanta Das. Enhanced oxidation resistance in nano-lamellar CoCrFeNiNbx (0.45 ≤ x ≤ 0.55) eutectic high entropy alloy during cyclic oxidation at 700–1100 °C. Corrosion Science, 2024, 240: 112497. DOI:10.1016/j.corsci.2024.112497 |
20. | Di Ouyang, Cheng Zhang, Renshun Chen, et al. The microcrack inhibition and mechanical properties of an in-situ synthesized refractory high-entropy alloy fabricated by additive manufacturing. Materials Science and Engineering: A, 2024, 913: 147071. DOI:10.1016/j.msea.2024.147071 |
21. | Haofei Sun, Emily Seto, Meifeng Li, et al. High-temperature oxidation behavior of transition metal complex concentrated alloys (TM-CCAs): a comprehensive review. Journal of Materials Chemistry A, 2024, 12(48): 33488. DOI:10.1039/D4TA06071G |
22. | Huaqing Yi, Xiangyun Yang, Yi Yang, et al. New insights in the oxidation behavior of (FeCoCrNi)94Al4Ti2Si high entropy alloys at 1100 °C. Corrosion Science, 2024, 227: 111673. DOI:10.1016/j.corsci.2023.111673 |
23. | Haofei Sun, Meifeng Li, Hao Zhang, et al. Phase transformation and diffusion in high-temperature oxidation of FeCrNi medium entropy alloy. Corrosion Science, 2024, 227: 111685. DOI:10.1016/j.corsci.2023.111685 |
24. | Longlong Liu, Jianlei Zhang, Changsheng Zhai, et al. Addressing challenges in high-temperature oxidation of ultra-supercritical boiler tubes via FeCoNiCrMo high-entropy alloy coatings. Surface and Coatings Technology, 2024, 477: 130351. DOI:10.1016/j.surfcoat.2023.130351 |
25. | Dihua Ouyang, Ruijin Mao, Langlang Zhang, et al. Study on the tensile properties of Al-Zn-Mg alloy based on molecular dynamics. Engineering Failure Analysis, 2024, 155: 107752. DOI:10.1016/j.engfailanal.2023.107752 |
26. | Qingwei Gao, Yingying Wang, Jianhong Gong, et al. High-temperature oxidation behaviors of Co-free Cr30Fe30Ni30Al5Ti5 dual-phase multi-component alloys with multi-scale nanoprecipitates. Materials Today Advances, 2024, 22: 100482. DOI:10.1016/j.mtadv.2024.100482 |
27. | A. B. Yurgin, A. A. Ruktuev, D. V. Lazurenko, et al. Effect of Cr3C2 Additives on the Structure and Properties of Cantor Alloy Coatings. Metal Science and Heat Treatment, 2024, 66(7-8): 406. DOI:10.1007/s11041-024-01064-8 |
28. | O. Zakir, O. Guler, R. Idouhli, et al. Enhanced photocatalytic abilities of innovative NbTaZrMoW high-entropy alloys (HEAs): a comparative analysis with its high entropy oxide (HEO) counterpart. Journal of Materials Science, 2024, 59(26): 12050. DOI:10.1007/s10853-024-09871-3 |
29. | Marián Palcut, Marián Drienovský, Pavol Priputen, et al. Oxidation resistance of AlCoFeNiCu high entropy alloys. Journal of Materials Research and Technology, 2024, 31: 1974. DOI:10.1016/j.jmrt.2024.06.185 |
30. | Xin Li, Chenglei Wang, Haiqing Qin, et al. Effect of Different Aging Times on the Microstructure and Properties of Fe24Co29Ni38Al3Ti6 High-Entropy Alloy. Journal of Materials Engineering and Performance, 2024. DOI:10.1007/s11665-024-09765-0 |
31. | Xiquan Jia, Zhenlin Xu, Yizhu He, et al. Oxidation Behavior of CoCrFeMnNi High-Entropy Alloy Fabricated by Selective Laser Melting. Metals and Materials International, 2023, 29(10): 2895. DOI:10.1007/s12540-023-01415-9 |
32. | Qi Zhao, Xi Huang, Zixiong Zhan, et al. Effect of alloying elements (Mn, Ti, and Mo) on the corrosion behavior of FeCoNiCr-based high entropy alloy in supercritical water. Corrosion Science, 2023, 220: 111291. DOI:10.1016/j.corsci.2023.111291 |
33. | Lingsheng Ke, Long Meng, Sheng Fang, et al. High-Temperature Oxidation Behaviors of AlCrTiSi0.2 High-Entropy Alloy Doped with Rare Earth La and Y. Crystals, 2023, 13(8): 1169. DOI:10.3390/cryst13081169 |
34. | Abdelhakim Bouissil, Sofiane Achache, Djallel Eddine Touaibia, et al. Properties of a new TiTaZrHfW( N) refractory high entropy film deposited by reactive DC pulsed magnetron sputtering. Surface and Coatings Technology, 2023, 462: 129503. DOI:10.1016/j.surfcoat.2023.129503 |
35. | Qinghua Wei, Bin Cao, Lucheng Deng, et al. Discovering a formula for the high temperature oxidation behavior of FeCrAlCoNi based high entropy alloys by domain knowledge-guided machine learning. Journal of Materials Science & Technology, 2023, 149: 237. DOI:10.1016/j.jmst.2022.11.040 |
36. | Na Xiao, Xu Guan, Dong Wang, et al. Impact of W alloying on microstructure, mechanical property and corrosion resistance of face-centered cubic high entropy alloys: A review. International Journal of Minerals, Metallurgy and Materials, 2023, 30(9): 1667. DOI:10.1007/s12613-023-2641-6 |
37. | Todd M. Butler, Michael J. Pavel, Mark L. Weaver. The effect of annealing on the microstructures and oxidation behaviors of AlCoCrFeNi complex concentrated alloys. Journal of Alloys and Compounds, 2023, 956: 170391. DOI:10.1016/j.jallcom.2023.170391 |
38. | Mikhail Slobodyan, Evgeniy Pesterev, Alexey Markov. Recent advances and outstanding challenges for implementation of high entropy alloys as structural materials. Materials Today Communications, 2023, 36: 106422. DOI:10.1016/j.mtcomm.2023.106422 |
39. | Teguh Widjajanto, Djarot B. Darmadi, Yudy Surya Irawan, et al. Comparative microstructure characteristics and properties of arc-sprayed Fe-based and HVOF-sprayed Ni-based coatings on ASME SA 210 C steel tube. Results in Engineering, 2023, 17: 100985. DOI:10.1016/j.rineng.2023.100985 |
40. | Zahid Hussain, Ateeq Ahmed, Bong-ju Lee, et al. Electrochemical Corrosion and High-Temperature Oxidation Behaviors of Three-dimensionally Interconnected Hexagonal Boron Nitride Reinforced CuNi composite. Arabian Journal for Science and Engineering, 2023, 48(9): 11861. DOI:10.1007/s13369-023-07605-2 |
41. | Bao-Zhen Wu, Te Zhu, Xing-Zhong Cao, et al. Investigation of the Oxidation Behavior of Cr20Mn17Fe18Ta23W22 and Microdefects Evolution Induced by Hydrogen Ions before and after Oxidation. Materials, 2022, 15(5): 1895. DOI:10.3390/ma15051895 |
42. | Zhuo Cheng, Shuize Wang, Guilin Wu, et al. Tribological properties of high-entropy alloys: A review. International Journal of Minerals, Metallurgy and Materials, 2022, 29(3): 389. DOI:10.1007/s12613-021-2373-4 |
43. | Indranil Roy, Chinedu Ekuma, Ganesh Balasubramanian. Examining the thermodynamic stability of mixed principal element oxides in AlCoCrFeNi high-entropy alloy by first-principles. Computational Materials Science, 2022, 213: 111619. DOI:10.1016/j.commatsci.2022.111619 |
44. | Youyou Zhang, Huibin Wu, Xinpan Yu, et al. Role of Cr in the High-Temperature Oxidation Behavior of Cr <sub>x</sub>MnFeNi High-Entropy Alloys at 800 °C in Air. SSRN Electronic Journal, 2022. DOI:10.2139/ssrn.4014287 |
45. | Youyou Zhang, Huibin Wu, Xinpan Yu, et al. Role of Cr in the high-temperature oxidation behavior of CrxMnFeNi high-entropy alloys at 800 °C in air. Corrosion Science, 2022, 200: 110211. DOI:10.1016/j.corsci.2022.110211 |
46. | Mingjie Wang, Xixi Huang, Shiping Wu, et al. Molecular dynamics simulations of tensile mechanical properties and microstructures of Al-4.5Cu alloy: the role of temperature and strain rate. Modelling and Simulation in Materials Science and Engineering, 2022, 30(4): 045004. DOI:10.1088/1361-651X/ac59d7 |
47. | M.L. Liang, C.L. Wang, C.J. Liang, et al. Microstructure and sliding wear behavior of FeCoNiCr0.8Al0.2 high-entropy alloy for different durations. International Journal of Refractory Metals and Hard Materials, 2022, 103: 105767. DOI:10.1016/j.ijrmhm.2021.105767 |
48. | Lingsheng Ke, chun lin, Mingtian Tan, et al. High-Temperature Oxidation Behaviors of Rare Earth La and Y Doped Alcrtisi0.2 High-Entropy Alloy. SSRN Electronic Journal, 2022. DOI:10.2139/ssrn.4161701 |
49. | Yi Zhang, Huadong Fu, Fanjie Zhou, et al. Revealing the effect of Al content on the oxidation of γ'-strengthened cobalt-based superalloys. Corrosion Science, 2022, 198: 110122. DOI:10.1016/j.corsci.2022.110122 |
HEA-1 | HEA-2 | HEA-3 | HEA-4 |
0.39 | 0.24 | 0.72 | 2.66 |
Point | Fe | Cr | Ni | Mn | Cu | Al | O |
A (Cr + Mn OL) | 0.3 ± 0.1 | 3.4 ± 0.2 | 0.2 ± 0.1 | 42.8 ± 0.3 | 0.2 ± 0.1 | 0 | 53.4 ± 0.3 |
B (Mn OL) | 0.2 ± 0.1 | 0.2 ± 0.1 | 0.2 ± 0.1 | 51.1 ± 0.2 | 0.2 ± 0.1 | 0 | 48.1 ± 0.3 |
C (Mn + Cu + Ni OL) | 0.2 ± 0.1 | 0.2 ± 0.1 | 1.9 ± 0.2 | 45.8 ± 0.4 | 15.3 ± 0.2 | 0 | 37.0 ± 0.4 |
D (Cr + Al + Mn OL) | 0.2 ± 0.1 | 1.2 ± 0.1 | 0.2 ± 0.1 | 47.1 ± 0.1 | 0.2 ± 0.1 | 6.1 ± 0.1 | 44.3 ± 0.3 |
E (Mn OL) | 0.1 ± 0.1 | 0.3 ± 0.1 | 0.2 ± 0.1 | 45.3 ± 0.3 | 0.2 ± 0.1 | 0.3 ± 0.1 | 53.7 ± 0.4 |
F (Mn + Cu + Ni OL) | 1.6 ± 0.1 | 0.1 ± 0.1 | 0.8 ± 0.1 | 54.8 ± 0.3 | 1.4 ± 0.1 | 0.3 ± 0.1 | 41.7 ± 0.3 |
Note: OL—Oxide layer. |