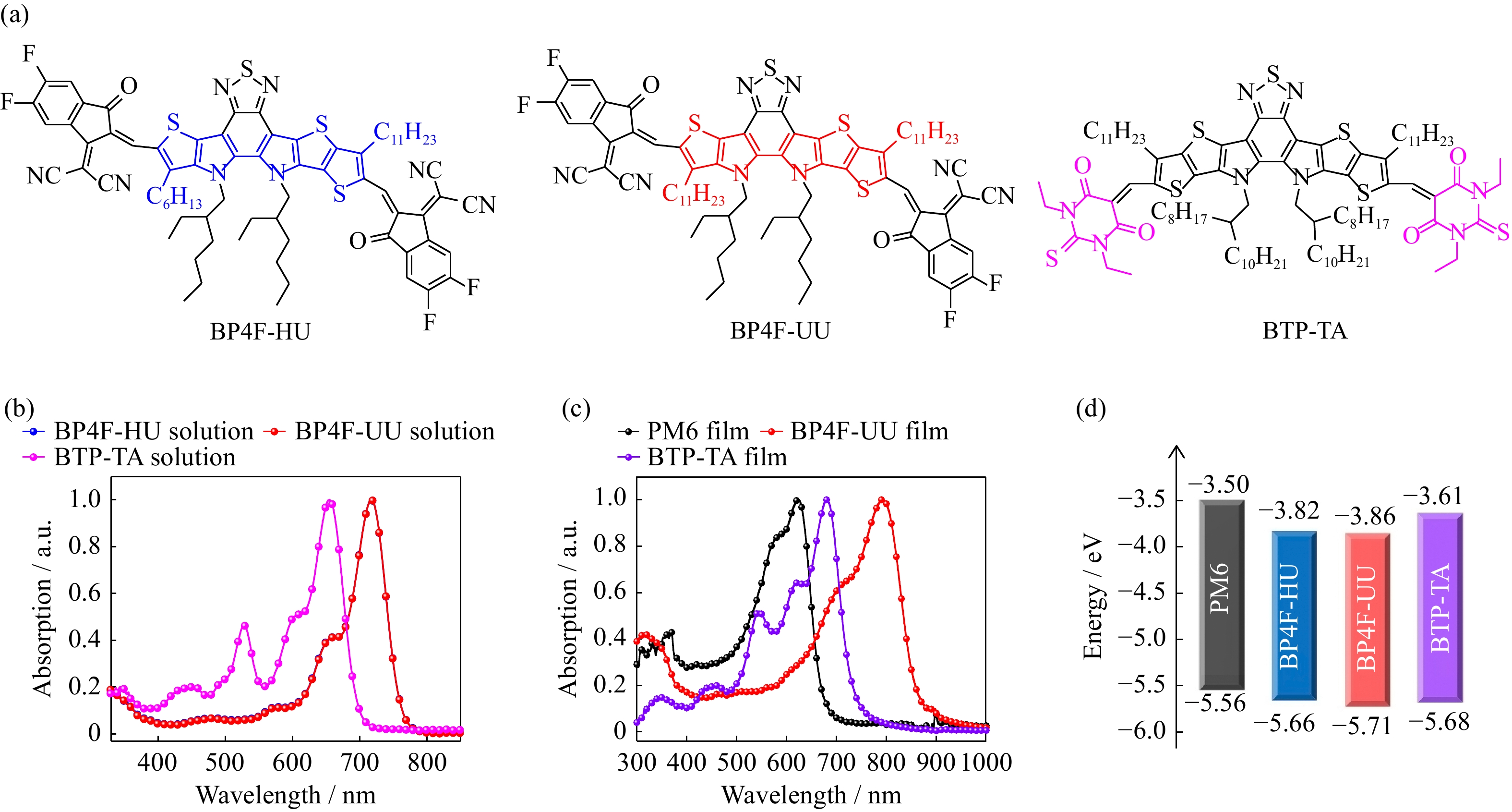
Cite this article as: | Shufang Li, Huilan Guan, Can Zhu, Chaoyuan Sun, Qingya Wei, Jun Yuan, and Yingping Zou, Alkyl chain modulation of asymmetric hexacyclic fused acceptor synergistically with wide bandgap third component for high efficiency ternary organic solar cells, Int. J. Miner. Metall. Mater., 31(2024), No. 7, pp.1713-1719. https://dx.doi.org/10.1007/s12613-024-2903-y |
Organic solar cells have witnessed rapid development during the past several years along with the emergence of A-DA'D-A small molecular acceptors (SMAs) and various device engineering [1–12]. Several strategies have been used to modify the molecular structure of the active materials, including the adjustment of conjugated fused rings [13–14], tuning of side chains [15–16], and regulation of the terminal group [17–18]. Device engineering made significant progress in ternary device [19–21], tandem device [22–24], electrode materials [25–26], additive, and solvent [27–28]. Up to date, the power conversion efficiency (PCE) of single junction organic solar cells (OSCs) has been close to 20% [29–30]. The synergy between fine molecular design and device engineering provides an effective approach to further realize the trade-off between open-circuit voltage (Voc), short-circuit current density (Jsc), and fill factor (FF) for developing highly efficient OSCs [31].
For the design and optimization of A-DA'D-A acceptors, modifying the rigid DA'D fused ring backbone is an available way to adjust absorption range, ionization energy, and π–π stacking behavior of the corresponding film. The common strategies are heteroatom substitution [32], asymmetric skeleton [33], extension of conjugated fused ring [34], etc. Among them, the investigation of asymmetric fused ring skeleton was found as an efficient method to endow SMAs with larger dipole moment and stronger intermolecular interactions, thus optimizing the molecule conformation and achieving the balance between solubility and crystallinity [2,35–37]. In addition, the outer side chain engineering at the terminal of the conjugated skeleton of A-DA'D-A SMAs is also a valid strategy to tune photovoltaic properties [38–39]. On the other hand, ternary device engineering has been proven to be a promising method for further advancing PCEs of OSCs in recent years. As the third component in ternary OSCs, the designed and synthesized wide bandgap materials possess multiple functions in blend film, such as expanding sunlight utilization [40], adjustable energy levels [41], improved charge transfer [42], and optimized film morphology [43], etc. In view of this, ternary devices have made remarkable progress in OSCs [29–30].
In this work, we firstly designed and synthesized A-DA'D-A type SMA BP4F-HU (Fig. 1(a)) with an asymmetric hexacyclic fused skeleton based on the previous works of our group [33,44]. The outer side chains of BP4F-HU at the terminal of the conjugated skeleton are hexyl and undecyl groups. Further, by fine-tuning the length of the outer side alkyl chain, a new small molecule with an asymmetric skeleton and two undecyl outer side chains was obtained, named BP4F-UU (Fig. 1(a)). Both the two small molecules were obtained by connecting a thieno [3.2-b] thiophene unit on one side of the electron-deficient core (BT) and a single thiophene unit on the other side to get a six-membered fused ring asymmetric skeleton, which could simplify the synthesis route and reduce the synthesis cost. The extension of the alkyl chain in the BP4F-UU molecule restricts the rotation of the end group and promotes the formation of more orderly molecular π–π stacking, thereby reducing energy disorder in the blend film. When fabricated into devices with PM6 as the polymer donor, BP4F-HU-based OSCs yield an optimal PCE of 15.31%, while BP4F-UU-based OSCs delivered a higher PCE of 15.82%. In order to further improve the photovoltaic efficiency of the device, we synthesized a new wide bandgap SMA, named BTP-TA (Fig. 1(a)), with a weakly electron-withdrawing terminal unit 1,3-diethyl-2-thiobarbituric acid, as the third component to fabricate ternary devices based on PM6:BP4F-UU system. BTP-TA can provide a perfect complementary absorption spectrum in the PM6:BP4F-UU system. In addition, due to the good overlap between the photoluminescence (PL) spectrum of BTP-TA and the absorption region of BP4F-UU, BTP-TA could achieve intermolecular energy transfer to BP4F-UU by fluorescence resonance energy transfer (FRET) pathway, which is an efficient way to reduce energy losses. At the same time, the high lowest unoccupied molecular orbital (LUMO) energy level of wide bandgap BTP-TA effectively improves the Voc in the device. Finally, the PM6:BP4F-UU:BTP-TA ternary device with 15wt% BTP-TA yields a significantly optimum PCE up to 17.83% with a synchronously elevated Voc of 0.905V, Jsc of 26.14 mA/cm2, FF of 75.38%. This work implies that the synergy effect of rational design of acceptor molecule structure and ternary device engineering is important to improving overall photovoltaic performance.
The indium tin oxide (ITO) glass substrates were cleaned sequentially under sonication with acetone, detergent, deionized water, and isopropyl alcohol, and then dried at 60°C in a baking oven overnight, followed by a 4-min oxygen plasma treatment. Then poly(3,4-ethylenedioxythiophene):poly(styrene sulfonate) (PEDOT:PSS) hole transport layer was filtered and spin-coated on top of the treated-ITO at 3000 r/min for 30 s and dried over 150°C for 20 min. The polymer donor:acceptors (D:A) mass ratio was 1:1.2 with a total concentration of 16 mg·mL−1, dissolved in chloroform (CF) and 1-fluoronaphthalene (FN) (0.8vol%). Subsequently, the solution was spin-cast at 3000 r/min for 30 s onto the PEDOT:PSS-coated substrates. The blend films were then transferred to the heating platform and annealed at 95°C for 10 min. A thin layer of poly[(9,9-bis(3'-((N,N-dimethyl)-N-ethylammonium)-propyl)-2,7-fluorene)-alt-2,7-(9,9-dioctylfluorene)] dibromide (PFN-Br) (0.5 mg/mL) was spin-coated on the top of the active layer with 3000 r/min for 30 s. Finally, the Ag (100 nm) electrode was evaporated onto the active layer under a vacuum of 10−4 Pa. The current density (J)–voltage (V) curves were measured on a computer-controlled Keithley 2400 source meter under 1 sun, AM 1.5 G spectra from a class solar simulator (Enlitech, China Taipei), and the light intensity was 100 mW/cm2 as calibrated by a China General Certification Center-certified reference monocrystal silicon cell (Enlitech). External quantum efficiency (EQE) values of the devices were measured using a QE-R3011 instrument (Enli Technology Co. Ltd., China Taipei) with a scan increment of 10 nm per point.
The relevant synthesis procedures were referenced from the previously published literature of our group [33,44], and the specific route is depicted in supplementary information (SI). All intermediates were determined by 1H nuclear magnetic resonance (NMR), and the final two small molecule acceptors, BP4F-HU and BP4F-UU, are evidenced by 1H NMR,13C NMR, and mass spectrum (MS). Both BP4F-HU and BP4F-UU show good solubility in general organic solvents like chloroform, dichloromethane, and chlorobenzene. The chemical structures of the two asymmetric SMAs are shown in Fig. 1(a), and the normalized absorption spectra are illustrated in Fig. 1(b) and (c). Due to the slight difference in molecular structure, BP4F-HU and BP4F-UU have an almost identical absorption spectrum with the solution absorption peak at 717 nm and neat film absorption peak at 790 nm. The absorption edge in the films is more than 100 nm red-shifted, reaching ~880 nm compared to the solution absorption spectrum, indicating strong aggregation of the molecular skeleton and strong π–π interactions in the solid-state, which is consistent with the predicted larger intrinsic dipole moment and strengthened intermolecular π–π stacking properties. To investigate the electrochemical properties of BP4F-HU and BP4F-UU, cyclic voltammetry was conducted in neat films, and the related results are shown in Fig. S1 with data collected in Table S1. From the results depicted in Fig. 1(d), the highest occupied molecular orbital (HOMO) and LUMO energy levels of BP4F-HU were estimated to be −5.66 and −3.82 eV, along with −5.71 and −3.86 eV for BP4F-UU, respectively. The energy levels of the two asymmetric acceptors both fit well with PM6, which is an important prerequisite for efficient photovoltaic device.
The conventional device configuration of ITO/PEDOT: PSS/active layer/PFN-Br/Ag was adopted to investigate the photovoltaic characteristics of PM6:BP4F-HU and PM6:BP4F-UU devices (Fig. 2). Detailed device fabrication process and characterizations were provided in the Supplemental Information (SI). The J–V curves of the corresponding binary OSCs were demonstrated in Fig. 2(e), and the photovoltaic statistical data was collected in Table 1. The optimized PM6:BP4F-UU devices delivered a PCE of 15.82% with Jsc of 24.03 mA·cm−2, FF of 74.95%, and Voc of 0.878 V, which is higher than the PM6:BP4F-HU devices of 15.31% (Jsc = 23.69 mA·cm−2, FF = 74.86%, and Voc = 0.863 V). The optimized PM6:BP4F-UU based OSC exhibits an improved Voc and Jsc compared to PM6:BP4F-HU, which could be ascribed to the stronger molecular stacking and optimal morphology, as discussed in details below.
To further improve the device performance, the third component BTP-TA, was added into the binary device of PM6:BP4F-UU. Considering the strong crystallinity of 1,3-diethyl-2-thiobarbituric acid end group [45], we selected a longer alkyl chains 2-octyldodecyl on the pyrrole ring N atom from the backbone of BTP-TA to balance the solubility and crystallinity. It is well known that optoelectronic properties of SMAs are related to its molecular structure, especially the intramolecular charge transfer between the fused backbone and terminal group. BTP-TA employs a weak electron-withdrawing unit, 1,3-diethyl-2-thiobarbituric acid, as the terminal group, exhibiting an absorption spectrum between 450–730 nm, The absorption range of BTP-TA effectively fills the absorption gap of PM6:BP4F-UU blend, which is favorable for enhancing photon utilization, and thus increasing the Jsc of the corresponding device. The upshifted LUMO energy level can be ascribed to the weaker electron-withdrawing ability of 1,3-diethyl-2-thiobarbituric acid end group. Fig. 2(d) depicts the working mechanism in ternary blends. The significantly higher LUMO energy level of BTP-TA compared to BP4F-UU is advantageous for higher Voc in ternary OSCs.
Active layer | Voc / V | Jsc / (mA·cm−2) | FF / % | PCE[a] / % | Eloss / eV |
PM6:BP4F-HU | 0.863 | 23.69 | 74.86 | 15.31 (14.94 ± 0.36) | 0.559 |
PM6:BP4F-UU | 0.878 | 24.03 | 74.95 | 15.82 (15.52 ± 0.34) | 0.544 |
PM6:BP4F-UU:BTP-TA | 0.905 | 26.14 | 75.38 | 17.83 (17.47 ± 0.33) | 0.517 |
Note: [a] Values in the bracket are the average PCEs from ten devices. |
Steady-state PL spectroscopy and differential scanning calorimetry (DSC) measurements were carried out to explore whether alloy-like phase was formed between BP4F-UU and BTP-TA [46–47]. As shown in Fig. 2(a), when excited at 550 nm, BTP-TA exhibited PL spectra ranging from 680–850 nm with an emission peak at 753 nm. The apparently observed spectral overlapping between BTP-TA PL spectra and BP4F-UU absorption spectra indicates the potential tendency of energy transfer from BTP-TA to BP4F-UU according to the Förster theory. Then PL spectra of BP4F-UU neat film and BP4F-UU:BTP-TA blend film with 15wt% BTP-TA was measured, as shown in Fig. 2(b). It was clear that BP4F-UU demonstrated an emission peak at 965 nm. When blended with BTP-TA, the emission peak of BP4F-UU exhibited a slightly blue shift to 941 nm with the simultaneously enhanced emission intensity, but the emission peak of BTP-TA was quenched completely, which indicated the occurrence of FRET from BTP-TA to BP4F-UU. The efficient energy transfer between the two acceptors provides an additional channel for photon utilization and energy loss reduction, which is beneficial for higher Jsc and Voc in ternary OSCs. Furthermore, the FRET effect demonstrates good compatibility and miscibility between BTP-TA and BP4F-UU to readily form an alloy phase. To further verify the formation of alloy-like composites, DSC measurement was conducted as shown in Fig. 2(c). BTP-TA delivers a sharp melting peak at 171°C, implying its strong crystallinity. BP4F-UU exhibits two melting points at 190 and 252°C, respectively, and then the melting points decreased to 159 and 243°C when adding 15wt% BTP-TA in total acceptors. The lowered melting points indicate that strong interaction existed in BP4F-UU and BTP-TA [48], and further verify good compatibility between BP4F-UU and BTP-TA to form an alloy-like phase.
The device fabrication details of ternary blend PM6:BP4F-UU:BTP-TA as the active layer was depicted in SI and the related results were provided in Table S2. J–V curves of the optimized ternary device were depicted in Fig. 2(e) and Fig. S2. When the weight ratio of BTP-TA in acceptors varies from 0 to 100%, a proportionally increasing Voc value was obtained in ternary OSCs, which delivers a universal characteristic of alloy-like phase and is consistent with the aforementioned results. However, the FF and Jsc showed a trend of first increasing and then decreasing, and obtained a maximum value when the weight ratio of BTP-TA was 15% in acceptors. Finally, the ternary device based on PM6:BP4F-UU:BTP-TA achieved the best PCE of 17.83% with 15wt% BTP-TA, resulting from the synchronously enhanced Voc, Jsc, and FF in comparison with corresponding binary OSCs. The EQEs and integrated current densities were conducted on optimal binary and ternary systems to elucidate the light response in devices, and the results were depicted in Fig. 2(f). Both binary and ternary devices exhibited an efficient photon-to-electron conversion process in the wavelength range of 560–810 nm over 80%. The integrated current densities calculated from EQE spectra are 23.48, 24.15, and 24.87 mA·cm−2 for the PM6:BP4F-HU, PM6:BP4F-UU, and ternary devices, respectively, which are consistent well with the Jsc obtained from the J–V measurements (within 5% error range). The energy loss (Eloss) was further explored for the best performance device of binary and ternary OSCs, and the results were shown in Table 1. Eloss is defined as (Eg−qVoc), where q is the elementary charge and the optical bandgap Eg is calculated from the EQE edge of the corresponding device. PM6:BP4F-HU based binary device exhibits an Eloss of 0.559 eV, while PM6:BP4F-UU with a lower value of 0.544 eV. The decreased Eloss in BP4F-UU binary devices can be ascribed to the extension of alkyl chain on beta position of thieno [3,2-b] thiophene, which restricts the rotation of end group and promotes to form more orderly molecular π–π stacking, thus reducing energy disorder [49–50]. Furthermore, the optimum ternary OSC shows an Eloss of 0.517 eV, which is relatively lower than those of BP4F-HU and BP4F-UU based binary devices. This result reveals that incorporating a medium bandgap acceptor with a higher LUMO energy level into the host binary blend is beneficial for reducing Eloss.
The dependence of the photocurrent density (Jph) on the effective voltage (Veff) was first studied to investigate the charge dissociation efficiency of the binary and optimized ternary devices. The photocurrent density (Jph) is calculated as Jph = JL − JD, where JL and JD mean the current densities under the condition of illumination and in the dark, respectively. The charge dissociation rate (Pdiss) can be calculated according to the equation of Pdiss = Jph/Jsat, where Jsat is the saturate photocurrent density at high Veff (defined as Veff = V0 − V, where V0 is the voltage when the efficient current density is zero, and V is the applied voltage). The related results are demonstrated in Fig. 3(a). The Pdiss values of 0.985, 0.986, and 0.993 were obtained under short-circuit conditions based on the optimized devices of PM6:BP4F-HU, PM6:BP4F-UU, and PM6:BP4F-UU:BTP-TA, respectively. It is obvious that the two binary devices exhibited approximate results but the ternary devices delivered a significantly improved Pdiss, suggesting charge extraction is more efficient in the ternary blend film. Therefore, the enhanced FF in ternary devices can be rationally ascribed to the improved charge dissociation and the higher charge collection efficiency.
To better understand charge transport performance of the optimized binary and ternary devices, the hole (μh) and electron (μe) mobility were tested by the space charge limited current (SCLC) method. The results were depicted in Fig. 3(b) and (c). BP4F-HU-based device displays a μe of 4.27 × 10−4 and μh of 6.94 × 10−4 cm2·V−1·s−1, while BP4F-UU-based device with an increased μe of 6.93 × 10−4 cm2·V−1·s−1 and μh of 8.10 × 10−4 cm2·V−1·s−1. The improved electron mobility in BP4F-UU can be attributed to the mitigated energy disorder facilitating faster carrier transport, conforming to the above discussion. Moreover, the optimized ternary device has a μe of 5.71 × 10−4 cm2·V−1·s−1 and μh of 5.95 × 10−4 cm2·V−1·s−1. It is obvious that ternary device has a more balanced electron and hole mobility (μe/μh = 1.04, while the binary devices based on BP4F-HU and BP4F-UU are 1.63 and 1.17, respectively), which partially contributed to its increased FF.
The dependence of voltage and current density on light intensity (Plight) were measured to investigate the charge recombination of binary and optimized ternary OSC devices. The slope of Voc ∝ lnPlight can be expressed as nkT/q (k is the Boltzmann constant, T is the absolute temperature, q is the elementary charge). When n is closer to 1, the trap-assisted recombination can be ignored [51]. As illustrated in Fig. S3, the PM6:BP4F-HU-based device achieved a maximum n value of 1.172, whereas the PM6:BP4F-UU-based device had an n value of 1.132. Notably, the optimized PM6:BP4F-UU:BTP-TA ternary OSC exhibited a reduced n value of only 1.098, indicating substantial suppression of trap-assisted recombination in this ternary system. The dependence of Jsc on light intensity can be described as Jsc ∝ Pαlight, where α closer to 1 means less bimolecular recombination [52]. The α value based on PM6:BP4F-HU, PM6:BP4F-UU, and PM6:BP4F-UU:BTP-TA is 0.977, 0.978, and 0.989, respectively, indicating the ternary device showed a minimum bimolecular recombination possibility, which is consistent with its improved Jsc. The improved charge separation, more balanced carrier transport, and reduced charge recombination together contributed to an overall enhanced photovoltaic performance of ternary OSCs.
To further explore the morphology of the blend films, we performed atomic force microscopy (AFM) and transmission electron microscopy (TEM) measurements. As depicted in Fig. 4, all the blends exhibited uniform and smooth surfaces, and nano-fiber domains can be observed in the phase images. The root-mean-square roughness (Rq) of the film based on PM6:BP4F-HU is slightly higher than the others, which was ascribed to the little bit poor solubility of BP4F-HU, which verify that the length of side chains has an important influence on film morphology. The ternary blend shows a minimum Rq of 1.11 nm, which was attributed to the optimized microstructure from the addition of the third component, thus beneficial for a higher FF. The TEM images were depicted in Fig. S4, it is clear that the optimized ternary blend film exhibited more uniform dark and light areas, which indicated the donor and acceptor forming a well-mixed nanoscale morphology [53].
In summary, two asymmetric hexacyclic acceptor, BP4F-HU and BP4F-UU, were designed and synthesized. The extended alkyl chains on the outer side of the BP4F-UU molecule played a pivotal role in improving charge mobility and minimizing energy loss within the device. Specifically, the PM6:BP4F-UU system achieved an efficiency of 15.82%, featuring a higher Voc of 0.878 V compared to the PM6:BP4F-HU device. After incorporating an absorption complementary third component BTP-TA into the optimal PM6:BP4F-UU binary device, the corresponding ternary device exhibited a high PCE of 17.83% with simultaneous enhanced Voc of 0.905 V, Jsc of 26.14 mA/cm2, and FF of 75.38%. The improvement in ternary device performance benefited from the good compatibility in two acceptors for the formation of alloy model, thus leading to broader photon utilization, optimal blend morphology, and reduced charge recombination.
This work was financially supported by the National Natural Science Foundation of China (Nos. 52125306 and 21875286).
There are no conflicts of interest to declare.
The online version contains supplementary material available at https://doi.org/10.1007/s12613-024-2903-y.
[1] |
L.L. Feng, J. Yuan, Z.Z. Zhang, et al., Thieno [3,2-b] pyrrolo-fused pentacyclic benzotriazole-based acceptor for efficient organic photovoltaics, ACS Appl. Mater. Interfaces, 9(2017), No. 37, p. 31985. DOI: 10.1021/acsami.7b10995
|
[2] |
M. Li, Y.Y. Zhou, J.Q. Zhang, J.S. Song, and Z.S. Bo, Tuning the dipole moments of nonfullerene acceptors with an asymmetric terminal strategy for highly efficient organic solar cells, J. Mater. Chem. A, 7(2019), No. 15, p. 8889. DOI: 10.1039/C8TA12530A
|
[3] |
K. Jiang, Q.Y. Wei, J.Y.L. Lai, et al., Alkyl chain tuning of small molecule acceptors for efficient organic solar cells, Joule, 3(2019), No. 12, p. 3020. DOI: 10.1016/j.joule.2019.09.010
|
[4] |
S. Liu, J. Yuan, W.Y. Deng, et al., High-efficiency organic solar cells with low non-radiative recombination loss and low energetic disorder, Nat. Photonics, 14(2020), No. 5, p. 300. DOI: 10.1038/s41566-019-0573-5
|
[5] |
C. Zhu, J. Yuan, F.F. Cai, et al., Tuning the electron-deficient core of a non-fullerene acceptor to achieve over 17% efficiency in a single-junction organic solar cell, Energy Environ. Sci., 13(2020), No. 8, p. 2459. DOI: 10.1039/D0EE00862A
|
[6] |
S.N. Bao, H. Yang, H.Y. Fan, et al., Volatilizable solid additive-assisted treatment enables organic solar cells with efficiency over 18.8% and fill factor exceeding 80, Adv. Mater., 33(2021), No. 48, art. No. 2105301. DOI: 10.1002/adma.202105301
|
[7] |
X.J. Chen, D. Wang, Z.K. Wang, et al., 18.02% efficiency ternary organic solar cells with a small-molecular donor third component, Chem. Eng. J., 424(2021), art. No. 130397. DOI: 10.1016/j.cej.2021.130397
|
[8] |
Y. Cui, Y. Xu, H.F. Yao, et al., Single-junction organic photovoltaic cell with 19% efficiency, Adv. Mater., 33(2021), No. 41, art. No. 2102420. DOI: 10.1002/adma.202102420
|
[9] |
L.Z. Liu, S.Y. Chen, Y.Y. Qu, et al., Nanographene–osmapentalyne complexes as a cathode interlayer in organic solar cells enhance efficiency over 18%, Adv. Mater., 33(2021), No. 30, art. No. 2101279. DOI: 10.1002/adma.202101279
|
[10] |
R.J. Ma, T. Yang, Y.Q. Xiao, et al., Air-processed efficient organic solar cells from aromatic hydrocarbon solvent without solvent additive or post-treatment: Insights into solvent effect on morphology, Energy Environ. Mater., 5(2022), No. 3, p. 977. DOI: 10.1002/eem2.12226
|
[11] |
G.U. Kim, C. Sun, D. Lee, et al., Effect of the selective halogenation of small molecule acceptors on the blend morphology and voltage loss of high-performance solar cells, Adv. Funct. Mater., 32(2022), No. 25, art. No. 2201150. DOI: 10.1002/adfm.202201150
|
[12] |
G.L. Cai, Z. Chen, X.X. Xia, et al., Pushing the efficiency of high open-circuit voltage binary organic solar cells by vertical morphology tuning, Adv. Sci., 9(2022), No. 14, art. No. 2200578. DOI: 10.1002/advs.202200578
|
[13] |
F. Lin, K. Jiang, W. Kaminsky, Z.L. Zhu, and A.K.Y. Jen, A non-fullerene acceptor with enhanced intermolecular π-core interaction for high-performance organic solar cells, J. Am. Chem. Soc., 142(2020), No. 36, p. 15246. DOI: 10.1021/jacs.0c07083
|
[14] |
Z.C. Zhou, W.R. Liu, G.Q. Zhou, et al., Subtle molecular tailoring induces significant morphology optimization enabling over 16% efficiency organic solar cells with efficient charge generation, Adv. Mater., 32(2020), No. 4, art. No. 1906324. DOI: 10.1002/adma.201906324
|
[15] |
Y. Cui, H.F. Yao, J.Q. Zhang, et al., Single-junction organic photovoltaic cells with approaching 18% efficiency, Adv. Mater., 32(2020), No. 19, art. No. 1908205. DOI: 10.1002/adma.201908205
|
[16] |
C. Li, J.D. Zhou, J.L. Song, et al., Non-fullerene acceptors with branched side chains and improved molecular packing to exceed 18% efficiency in organic solar cells, Nat. Energy, 6(2021), No. 6, p. 605. DOI: 10.1038/s41560-021-00820-x
|
[17] |
Z.H. Luo, R.J. Ma, T. Liu, et al., Fine-tuning energy levels via asymmetric end groups enables polymer solar cells with efficiencies over 17%, Joule, 4(2020), No. 6, p. 1236. DOI: 10.1016/j.joule.2020.03.023
|
[18] |
H. Chen, H.J. Lai, Z.Y. Chen, et al., 17.1%-efficient eco-compatible organic solar cells from a dissymmetric 3D network acceptor, Angew. Chem. Int. Ed., 60(2021), No. 6, p. 3238. DOI: 10.1002/anie.202013053
|
[19] |
F. Liu, L. Zhou, W.R. Liu, et al., Organic solar cells with 18% efficiency enabled by an alloy acceptor: A two-in-one strategy, Adv. Mater., 33(2021), No. 27, art. No. 2100830. DOI: 10.1002/adma.202100830
|
[20] |
Y.H. Cai, Y. Li, R. Wang, et al., A well-mixed phase formed by two compatible non-fullerene acceptors enables ternary organic solar cells with efficiency over 18.6%, Adv. Mater., 33(2021), No. 33, art. No. 2101733. DOI: 10.1002/adma.202101733
|
[21] |
T. Zhang, C.B. An, P.Q. Bi, et al., A thiadiazole-based conjugated polymer with ultradeep HOMO level and strong electroluminescence enables 18.6% efficiency in organic solar cell, Adv. Energy Mater., 11(2021), No. 35, art. No. 2101705. DOI: 10.1002/aenm.202101705
|
[22] |
G.C. Liu, R.X. Xia, Q.R. Huang, et al., Tandem organic solar cells with 18.7% efficiency enabled by suppressing the charge recombination in front sub-cell, Adv. Funct. Mater., 31(2021), No. 29, art. No. 2103283. DOI: 10.1002/adfm.202103283
|
[23] |
J.Q. Wang, Z. Zheng, Y.F. Zu, et al., A tandem organic photovoltaic cell with 19.6% efficiency enabled by light distribution control, Adv. Mater., 33(2021), No. 39, art. No. 2102787. DOI: 10.1002/adma.202102787
|
[24] |
Z. Zheng, J.Q. Wang, P.Q. Bi, et al., Tandem organic solar cell with 20.2% efficiency, Joule, 6(2022), No. 1, p. 171. DOI: 10.1016/j.joule.2021.12.017
|
[25] |
X.J. Zheng, L.J. Zuo, F. Zhao, et al., High-efficiency ITO-free organic photovoltaics with superior flexibility and upscalability, Adv. Mater., 34(2022), No. 17, art. No. 2200044. DOI: 10.1002/adma.202200044
|
[26] |
J. Yao, B.B. Qiu, Z.G. Zhang, et al., Cathode engineering with perylene-diimide interlayer enabling over 17% efficiency single-junction organic solar cells, Nat. Commun., 11(2020), No. 1, art. No. 2726. DOI: 10.1038/s41467-020-16509-w
|
[27] |
J. Lv, H. Tang, J.M. Huang, et al., Additive-induced miscibility regulation and hierarchical morphology enable 17.5% binary organic solar cells, Energy Environ. Sci., 14(2021), No. 5, p. 3044. DOI: 10.1039/D0EE04012F
|
[28] |
X.P. Xu, L.Y. Yu, H. Yan, R.P. Li, and Q. Peng, Highly efficient non-fullerene organic solar cells enabled by a delayed processing method using a non-halogenated solvent, Energy Environ. Sci., 13(2020), No. 11, p. 4381. DOI: 10.1039/D0EE02034F
|
[29] |
L. Zhu, M. Zhang, J.Q. Xu, et al., Single-junction organic solar cells with over 19% efficiency enabled by a refined double-fibril network morphology, Nat. Mater., 21(2022), No. 6, p. 656. DOI: 10.1038/s41563-022-01244-y
|
[30] |
L.L. Zhan, S.C. Yin, Y.K. Li, et al., Multiphase morphology with enhanced carrier lifetime via quaternary strategy enables high-efficiency thick-film, and large-area organic photovoltaics, Adv. Mater., 34(2022), No. 45, art. No. 2206269. DOI: 10.1002/adma.202206269
|
[31] |
J.Q. Zhang, H.S. Tan, X.G. Guo, A. Facchetti, and H. Yan, Material insights and challenges for non-fullerene organic solar cells based on small molecular acceptors, Nat. Energy, 3(2018), No. 9, p. 720. DOI: 10.1038/s41560-018-0181-5
|
[32] |
Z.Z. Zhang, Y.W. Li, G.L. Cai, Y.H. Zhang, X.H. Lu, and Y.Z. Lin, Selenium heterocyclic electron acceptor with small urbach energy for as-cast high-performance organic solar cells, J. Am. Chem. Soc., 142(2020), No. 44, p. 18741. DOI: 10.1021/jacs.0c08557
|
[33] |
F.F. Cai, H.J. Peng, H.G. Chen, et al., An asymmetric small molecule acceptor for organic solar cells with a short circuit current density over 24 mA·cm−2, J. Mater. Chem. A, 8(2020), No. 31, p. 15984. DOI: 10.1039/D0TA01636E
|
[34] |
W. Gao, H.T. Fu, Y.X. Li, et al., Asymmetric acceptors enabling organic solar cells to achieve an over 17% efficiency: Conformation effects on regulating molecular properties and suppressing nonradiative energy loss, Adv. Energy Mater., 11(2021), No. 4, art. No. 2003177. DOI: 10.1002/aenm.202003177
|
[35] |
Y.Z. Chen, F.J. Bai, Z.X. Peng, et al., Asymmetric alkoxy and alkyl substitution on nonfullerene acceptors enabling high-performance organic solar cells, Adv. Energy Mater., 11(2021), No. 3, art. No. 2003141. DOI: 10.1002/aenm.202003141
|
[36] |
L.Y. Su, H.H. Huang, Y.C. Lin, et al., Enhancing long-term thermal stability of non-fullerene organic solar cells using self-assembly amphiphilic dendritic block copolymer interlayers, Adv. Funct. Mater., 31(2021), No. 4, art. No. 2005753. DOI: 10.1002/adfm.202005753
|
[37] |
J.S. Song and Z.S. Bo, Asymmetric molecular engineering in recent nonfullerene acceptors for efficient organic solar cells, Chin. Chem. Lett., 34(2023), No. 10, art. No. 108163. DOI: 10.1016/j.cclet.2023.108163
|
[38] |
Z.H. Luo, Y. Gao, H.J. Lai, et al., Asymmetric side-chain substitution enables a 3D network acceptor with hydrogen bond assisted crystal packing and enhanced electronic coupling for efficient organic solar cells, Energy Environ. Sci., 15(2022), No. 11, p. 4601. DOI: 10.1039/D2EE01848A
|
[39] |
X. Zhang, C.Q. Li, L.Q. Qin, et al., Side-chain engineering for enhancing the molecular rigidity and photovoltaic performance of noncovalently fused-ring electron acceptors, Angew. Chem. Int. Ed., 60(2021), No. 32, p. 17720. DOI: 10.1002/anie.202106753
|
[40] |
Y.L. Yin, L.L. Zhan, M. Liu, et al., Boosting photovoltaic performance of ternary organic solar cells by integrating a multi-functional guest acceptor, Nano Energy, 90(2021), art. No. 106538. DOI: 10.1016/j.nanoen.2021.106538
|
[41] |
L.L. Zhan, S.X. Li, Y.K. Li, et al., Desired open-circuit voltage increase enables efficiencies approaching 19% in symmetric-asymmetric molecule ternary organic photovoltaics, Joule, 6(2022), No. 3, p. 662. DOI: 10.1016/j.joule.2022.02.001
|
[42] |
X.W. Guo, D.Q. Li, Y.X. Zhang, et al., Understanding the effect of N2200 on performance of J71:ITIC bulk heterojunction in ternary non-fullerene solar cells, Org. Electron., 71(2019), p. 65. DOI: 10.1016/j.orgel.2019.05.004
|
[43] |
J. Lee, S.M. Lee, S. Chen, et al., Organic photovoltaics with multiple donor–acceptor pairs, Adv. Mater., 31(2019), No. 20, art. No. 1804762. DOI: 10.1002/adma.201804762
|
[44] |
F.F. Cai, C. Zhu, J. Yuan, et al., Efficient organic solar cells based on a new “Y-series” non-fullerene acceptor with an asymmetric electron-deficient-core, Chem. Commun., 56(2020), No. 31, p. 4340. DOI: 10.1039/C9CC10076H
|
[45] |
L.G. Xiao, M.A. Kolaczkowski, Y.G. Min, and Y. Liu, Substitution effect on thiobarbituric acid end groups for high open-circuit voltage non-fullerene organic solar cells, ACS Appl. Mater. Interfaces, 12(2020), No. 37, p. 41852. DOI: 10.1021/acsami.0c11828
|
[46] |
N.Y. Doumon, L.L. Yang, and F. Rosei, Ternary organic solar cells: A review of the role of the third element, Nano Energy, 94(2022), art. No. 106915. DOI: 10.1016/j.nanoen.2021.106915
|
[47] |
L.C. Chang, M. Sheng, L.P. Duan, and A. Uddin, Ternary organic solar cells based on non-fullerene acceptors: A review, Org. Electron., 90(2021), art. No. 106063. DOI: 10.1016/j.orgel.2021.106063
|
[48] |
Y.J. Cheng, B. Huang, X.X. Huang, et al., Oligomer-assisted photoactive layers enable >18% efficiency of organic solar cells, Angew. Chem. Int. Ed., 61(2022), No. 21, art. No. e202200329. DOI: 10.1002/anie.202200329
|
[49] |
Z.P. Yu, Z.X. Liu, F.X. Chen, et al., Simple non-fused electron acceptors for efficient and stable organic solar cells, Nat. Commun., 10(2019), art. No. 2152. DOI: 10.1038/s41467-019-10098-z
|
[50] |
J. Yuan, C.J. Zhang, H.G. Chen, et al., Understanding energetic disorder in electron-deficient-core-based non-fullerene solar cells, Sci. China Chem., 63(2020), No. 8, p. 1159. DOI: 10.1007/s11426-020-9747-9
|
[51] |
B. Qiu, Z. Chen, S. Qin, et al., Highly efficient all-small-molecule organic solar cells with appropriate active layer morphology by side chain engineering of donor molecules and thermal annealing, Adv. Mater., 32(2020), No. 21, art. No. 1908373. DOI: 10.1002/adma.201908373
|
[52] |
S.X. Li, L.L. Zhan, C.K. Sun, et al., Highly efficient fullerene-free organic solar cells operate at near zero highest occupied molecular orbital offsets, J. Am. Chem. Soc., 141(2019), No. 7, p. 3073. DOI: 10.1021/jacs.8b12126
|
[53] |
J.N. Song, M. Zhang, M. Yuan, Y.H. Qian, Y.M. Sun, and F. Liu, Morphology characterization of bulk heterojunction solar cells, Small Methods, 2(2018), No. 3, art. No. 1700229. DOI: 10.1002/smtd.201700229
|
[1] | Xu Zhao, Naitao Gao, Shengcheng Wu, Shaozhen Li, Sujuan Wu. Enhancing performance of low-temperature processed CsPbI2Br all-inorganic perovskite solar cells using polyethylene oxide-modified TiO2 [J]. International Journal of Minerals, Metallurgy and Materials, 2024, 31(4): 786-794. DOI: 10.1007/s12613-023-2742-2 |
[2] | Xu Zhao, Shoudeng Zhong, Shuqi Wang, Shaozhen Li, Sujuan Wu. Potassium thiocyanate additive for PEDOT:PSS layer to fabricate efficient tin-based perovskite solar cells [J]. International Journal of Minerals, Metallurgy and Materials, 2023, 30(12): 2451-2458. DOI: 10.1007/s12613-023-2738-y |
[3] | Xiaomeng Li, Pengcheng Jia, Fanwen Meng, Xingyu Zhang, Yang Tang, Bo Song, Chang Gao, Liang Qin, Feng Teng, Yanbing Hou. Propylamine hydrobromide passivated tin-based perovskites to efficient solar cells [J]. International Journal of Minerals, Metallurgy and Materials, 2023, 30(10): 1965-1972. DOI: 10.1007/s12613-023-2604-y |
[4] | Xiang Li, Baozhong Ma, Chengyan Wang, Die Hu, Yingwei Lü, Yongqiang Chen. Recycling and recovery of spent copper–indium–gallium–diselenide (CIGS) solar cells: A review [J]. International Journal of Minerals, Metallurgy and Materials, 2023, 30(6): 989-1002. DOI: 10.1007/s12613-022-2552-y |
[5] | Zhongbao Que, Liang Chu, Shuaibo Zhai, Yifei Feng, Chen Chen, Wei Liu, Ruiyuan Hu, Jing Hu, Xing’ao Li. Self-assembled TiO2 hole-blocking layers for efficient perovskite solar cells [J]. International Journal of Minerals, Metallurgy and Materials, 2022, 29(6): 1280-1285. DOI: 10.1007/s12613-021-2361-8 |
[6] | Jiuyao Du, Mengqi Zhang, Jianjun Tian. Controlled crystal orientation of two-dimensional Ruddlesden–Popper halide perovskite films for solar cells [J]. International Journal of Minerals, Metallurgy and Materials, 2022, 29(1): 49-58. DOI: 10.1007/s12613-021-2341-z |
[7] | Huan-yu Zhang, Rui Li, Wen-wu Liu, Mei Zhang, Min Guo. Research progress in lead-less or lead-free three-dimensional perovskite absorber materials for solar cells [J]. International Journal of Minerals, Metallurgy and Materials, 2019, 26(4): 387-403. DOI: 10.1007/s12613-019-1748-2 |
[8] | Sheng-jun Li, Yuan Lin, Wei-wei Tan, Jing-bo Zhang, Xiao-wen Zhou, Jin-mao Chen, Zeng Chen. Preparation and performance of dye-sensitized solar cells based on ZnO-modified TiO2 electrodes [J]. International Journal of Minerals, Metallurgy and Materials, 2010, 17(1): 92-97. DOI: 10.1007/s12613-010-0116-z |
[9] | Shu-huei Hsieh, Wen-jauh Chen, Pei-i Wei, Jiing-herng Lee. Synthesis of the CuInSe2 thin film for solar cells using the electrodeposition technique and Taguchi method [J]. International Journal of Minerals, Metallurgy and Materials, 2009, 16(1): 101-107. DOI: 10.1016/S1674-4799(09)60017-0 |
[10] | Takenori Notoya. Localized Corrosion in Copper Tubes by Volatile Organic Substance [J]. International Journal of Minerals, Metallurgy and Materials, 1999, 6(2): 129-132. |
Active layer | Voc / V | Jsc / (mA·cm−2) | FF / % | PCE[a] / % | Eloss / eV |
PM6:BP4F-HU | 0.863 | 23.69 | 74.86 | 15.31 (14.94 ± 0.36) | 0.559 |
PM6:BP4F-UU | 0.878 | 24.03 | 74.95 | 15.82 (15.52 ± 0.34) | 0.544 |
PM6:BP4F-UU:BTP-TA | 0.905 | 26.14 | 75.38 | 17.83 (17.47 ± 0.33) | 0.517 |
Note: [a] Values in the bracket are the average PCEs from ten devices. |